All published articles of this journal are available on ScienceDirect.
SARS-CoV-2 and Therapeutic Approaches
Abstract
In 2019, the Severe Acute Respiratory Syndrome Coronavirus (SARS-CoV-2) was first reported in Wuhan, China, affecting the population all over the globe. This ongoing pandemic is creating major havoc and has already taken the lives of many people. It has become really difficult to control the spread of the virus because of its high rate of multiplication in the host and transmission. The virus spreads by the means of droplets or direct contact with the infected person, causing pneumonia-like symptoms, such as fever, dry cough, throat pain, and breathlessness. This review presents the indications, mechanism of action, administration, and adverse effects of some antiviral, antibiotic, and immunomodulatory drugs, that are currently employed in the treatment of SARS-CoV-2. It also presents the effects and benefits of drugs on an infected person, when co-administered together.
1. INTRODUCTION
SARS-CoV-2 was initially reported in Wuhan, China, in December 2019 and is now causing major havoc all over the world as a global pandemic [1]. This ongoing pandemic has already crossed a number of 158 million confirmed cases and more than 3.3 million confirmed deaths [2]. Initially, in 2003, a similar major outbreak, SARS-CoV, was observed in almost five continents with a mortality rate of more than 10% [3]. A few years later, the Middle East Respiratory Syndrome Coronavirus (MERS-CoV) broke out in Arabian Peninsula in 2012, with a mortality rate of 35% [3].
1.1. Structure of SARS-CoV-2
CoVs are enveloped viruses with a positive ssRNA genome that has 16 non-structural proteins and 4 structural proteins, namely, Spike protein (S), Envelope protein (P), Membrane protein (M), and Nucleoprotein (N) [4]. These CoVs are also further divided into 4 genera [5]:
- Alpha CoV
- Beta CoV
- Gamma CoV
- Delta CoV
All these genera are generated as a result of genetic mutation in the original virus. Coronaviruses belong to the family of Coronaviridae [6]. They target the Angiotensin-converting enzyme-2 (ACE2) receptors inside the body and bind with them to form a complex that further leads to the replication of the virus [7] (Fig. 1).
1.2. Etiology
The spread of the virus could not be stopped to date because of its high rate of multiplication in the host and transmission [8]. After the onset of the disease, influenza-like symptoms are observed in infected patients after 5 days [2]. There is an effective immune response mediated by T and B lymphocytes in the asymptomatic population.
The clinical manifestations of SARS-CoV-2 include [4]:
- Fever
- Dry cough
- Throat pain
- SpO2 < 95%
- Breathlessness
- Loss of taste and smell
- Pneumonia in severe cases
Since it was a new virus, the pathophysiology of replication, was not clear initially, and hence the development of drugs that worked against this virus took a very long time [9]. As bacteria are whole living cells with all possible metabolic pathways, they produce various targets to attack.
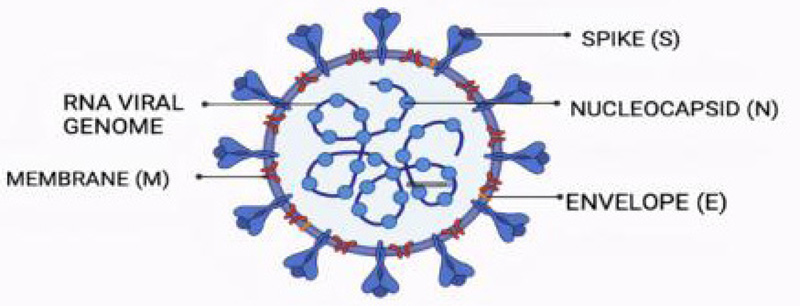
On the contrary, viruses are trickier as they reside inside the living cells of proteins, so they do not cause a direct attack. As a virus depends on many processes in a cell, various classes of drugs that attack proteins are tried to combat its multiplication. The conventional way of drug discovery is a lengthy process. So, the researchers are targeting the human immune system with interference RNA (iRNA) or vaccines. As per recent trials, the antivirals are proving less efficacious in the management of COVID -19, so various categories of drugs are used in combinations [10]. Even today, clinical trials and experiments are going on to develop newer and safer drugs that are more effective against this virus [11].
2. DRUGS PROJECTED IN COVID-19 TREATMENT
2.1. Antivirals
2.1.1. Remdesivir Indications
Remdesivir (GS-5734) is a nucleotide analog prodrug marketed by the brand name of Remdac (Zydus, Cadila) [12]. It is specifically used for hospitalized patients [13]. It works by reducing the time of recovery, and the retrieval starts in 10 days compared to a placebo, in which it is 15 days [14]. Different clinical reports showed mixed results.
It is more effective when given in combination with other drugs, such as Baricitinib, which is a selective inhibitor of JAK 1 and JAK 2 (Janus Kinase) and has an inhibitory effect on SARS-CoV-2 endocytosis [14].
Remdesivir was actually being tested for the Ebola virus in USA based company but was found ineffective [15, 16]. So, the scientists repurposed it for COVID patients. Currently, Veklury (remdesivir) is the only approved antiviral drug by US Food and Drug Administration (FDA) for the SARS-CoV-2 [9] (Table 1). However, the results of various clinical trials state that the drug does not show a significant difference in the improvement of condition compared to the placebo treatment or the standard treatment given to the patients [17, 18]. In the study published by Wang et al., 596 patients were clinically observed, out of which 584 received the Remdesivir treatment. The findings were similar to the standard treatment given to the patients [18].
2.1.1.1. Mechanism of Action
Remdesivir is a broad-spectrum antiviral drug that acts by inhibiting RdRp and targets the viral genome replication process [19]. RdRp synthesizes gRNA and sgRNA, which leads to viral replication [13]. RdRp is selectively expressed in viruses and hence is pointed out as an antiviral target [12-14]. Since remdesivir is an adenosine prolog, it competes with ATP (Adenosine Triphosphate) for incorporation [13, 20, 21]. It is a prodrug and is metabolized by host cells to its pharmacological active analog- GS- 441524 [20, 21]. This active analog inside the body competes with ATP for binding to the RdRp complex [13, 22]. Thus, it prevents the processing of new viral genome by competitive inhibition inside the cell [13]. Hence, the probable mechanism of action of the drug is the delayed chain termination which can be caused by the various possible pathways like structural alterations in the newly synthesized RNA [22].
S.No. | Name of Drug | Indications and Mechanism of Action |
---|---|---|
1. | Remdesivir | Antiviral; RdRp (RNA dependent RNA polymerase) inhibitor. |
2. | Favipiravir | Antiviral; RdRp inhibitor. |
3. | Ivermectin | Antiparasitic, antiviral, anticancer, and antibacterial; Inhibits Importin (IMP) alpha/beta mediated nuclear transport of viral proteins. |
4. | Chloroquine and Hydroxychloroquine | Anti-inflammatory, anti-malarial, and antiviral; Inhibits viral glycosylation and alters the pH. |
5. | Doxycycline | Antibiotic and antiviral; Inhibits the protein synthesis of viral cells by binding to specific mRNA. |
6. | Azithromycin | Antibiotic, antiviral, and anti-inflammatory; Prevents the translation of mRNA by binding itself to 50S ribosomal subunit. |
7. | Lopinavir | Antiviral; Aspartate protease inhibitor. |
8. | Amoxicillin | Antibiotic: Inhibits protein synthesis. |
9. | Ascorbic acid / Vit. C | Anti-oxidant; Acts by inflammation suppression and immune-regulation. |
10. | Colchicine | Analgesic and anti-inflammatory; Inhibits the chemotaxis of monocytes and neutrophils. |
11. | Dexamethasone | Anti-inflammatory and immune-suppressive; limits the production and damaging effects of cytokines. |
12. | Tocilizumab | Cytokine (IL-6) blocking agent; used to treat Cytokine Release Syndrome (CRS). |
13. | Zinc | Anti-inflammatory and anti-oxidant; Acts by decreasing cytokine production. |
14. | Vitamin D3 | Immuno-modulatory and anti-inflammatory; Acts by decreasing cytokine production. |
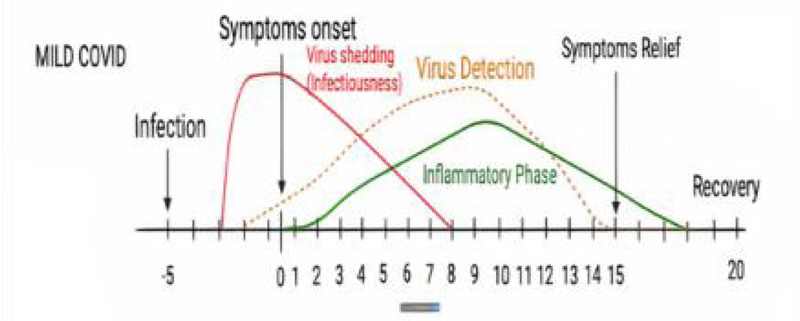
2.1.1.2. Administration
It is given intravenously because of the widespread distribution of GS-441524, providing 100% bioavailability [14, 21].
As per the severity of the patient, the dose is adjusted for 4-10 days. Generally, it is administered intravenously (i.v.) for 10 days, starting with a loading dose of 200 mg on the first day, subsequently maintenance doses of 100 mg for the next 9 days [14, 21]. It has been found that remdesivir injection showed accelerated recovery in hospitalized patients, which was consistent with the preliminary findings [13].
It is metabolized by Cytochrome 450 (CYP450) in the liver by Carboxylesterase 1 and eliminated in the urine as nucleoside metabolite – GS441524 [20].
2.1.1.3. Adverse Effects
The side effects of the drug are still not clear [13]. However, some that are observed affect the various organs of the body (such as the heart, liver, gastrointestinal tract (GIT), brain, and lungs) and are hypotension, anemia, dyspnea, septic shock, nausea, vomiting, cardiac arrest, poor appetite, insomnia, pyrexia, rash, delirium, mental confusion, and many more [16, 20]. Further studies are going on to find out more details in this regard (Fig. 2).
2.1.2. Lopinavir
2.1.2.1. Indications
Lopinavir is the second-line treatment, an anti-retroviral drug, that is used especially in the clinical treatment of HIV-1 [23]. It has been repurposed for SARS-CoV-2 and is given to COVID-positive patients to decrease the viral load [24, 25].
According to the study conducted between 19th March, 2020 and 29th June, 2020 by the Recovery Collaborative group, a total of 1616 patients were randomly given lopinavir-ritonavir treatment and 3424 received the standard care, out of which approximately 23% of patients in the treatment group of lopinavir-ritonavir and 22% patients in the standard group died [26]. Hence, the results of clinical trials on Lopinavir indicated that the drug was not completely efficacious in COVID-19 patients, and the mortality rate in both standard and test populations did not vary much, though it might have small benefits due to its proposed mechanism of action [25, 27].
2.1.2.2. Mechanism of Action
Though the mechanism of action is still unclear, it is clinically reported that the drug can inhibit the 3-chymotrypsin-like protease of SARS-CoV-1 and MERS and papain-like protease of SARS-CoV-2 [14]. It is a potent aspartate protease inhibitor designed in a way to compliment the C2 axis of the enzyme active site [28].
2.1.2.3. Administration
Lopinavir is given orally to the patients with a dose ranging between 100-400 mg, twice daily [29, 30]. It gives promising results when given at a very early stage of covid infection, reduces body temperature, mortality rate, and inflammation, and shortens the duration of ICU stays [23].
When co-administered with Ribavirin in the treatment of covid, it improves the condition more effectively as well as with interferon beta 1 b also [14]. It has been observed that early intake of lopinavir with interferon increased the viral shedding and improved conditions, especially in children [28]. When combined with Ritonavir, the plasma half-life was increased by inhibition of CYP450 [24, 30]. After its administration, the patient’s clinical reports result in abnormal WBC, lymphocytes, CPR, and normal PLT count [23, 28].
Lopinavir shows effective drug-drug interactions with Azithromycin, Chloroquine, and Remdesivir, inducing severe toxicity and hence should be used with caution [28].
It is metabolized in the liver by CYP3A4 and is generally excreted with feces and urine; Phenytoin induces the clearance of the drug via CYP3A4 induction [3, 28]. Although it is generally very well-tolerated by the body and shows greater patient compliance, some adverse effects are also reported, like diarrhea, hypersensitivity, nausea, vomiting, abdominal discomfort, and QT prolongation (in ECG); respiratory failure and acute kidney injury are also observed [29, 30]. Still, more research is required to further understand whether the drug decreases the viral load or duration of viral RNA detectability [24].
2.1.3. Ivermectin
2.1.3.1. Indications
Ivermectin is a broad-spectrum endo/ectoantiparasitic drug that belongs to the Avermectin family and is isolated from Streptomyces avermitilis [31, 32]. Being a USFDA-approved drug, repurposing it for SARS-CoV-2 will not be a problem.
It is the key drug used in the treatment of onchocerciasis and is the safest and the most effective drug against various RNA and DNA viruses, such as HIV 1, tick-borne encephalitis virus, yellow fever virus, influenza virus, West Nile virus, porcine-circa virus, parvovirus, Zika virus, and pseudorabies virus [32]. It is a potent inhibitor of the yellow fever virus and a weak inhibitor of flavivirus [33].
2.1.3.2. Mechanism of Action
The exact mechanism of action of ivermectin against SARS-CoV-2 is still unknown [34]. It is assumed to work by inhibiting the IMP alpha/beta1 mediated nuclear transport, thereby blocking the nuclear trafficking of viral proteins [31]. This binding of ivermectin to IMP alpha/beta heterodimer leads to changes in the stability of the structure and so prevents viral protein to bind to IMP alpha/beta 1 [35]. Hence, it shows antiviral activity by blocking the viral proteins to enter the nucleus [35].
It works against Vero- hSLAM cells within 2 hours of viral attack [31, 32]. There is a great reduction in viral RNA after 48 hours as it inhibits viral replication in the body, but its effect is unpronounced in hospitalized patients [33].
It also works as an antiparasitic drug by paralyzing and killing them [32]. For flavivirus, it targets the non-structural protein 3 helicase (NS3 helicase), and in HIV 1, the non-structural protein 5 (NS5), which is again dependent on alpha/beta nuclear transport [32].
2.1.3.3. Administration
It is given orally (i.e., 150 micrograms/kg), generally on an empty stomach, in the form of tablets to lower the mortality rate [34]. When it is used in combination with hydroxychloroquine, it produces a synergistic inhibitory effect on SARS-CoV-2 [36]. The hydroxychloroquine stops the viral entry in the cell and ivermectin stops viral replication inside the cell [36]. If given in the early stages of infection, they limit the viral load and prevent severe disease progression [33] (Fig. 3).
2.1.4. Favipiravir
2.1.4.1. Indications
Favipiravir, marketed as FabiFlu, is a broad-spectrum antiviral drug [8]. It was initially approved in Japan for the treatment of influenza [6]. It is also effective against the Ebola virus, Lassa virus, and Rabies virus [37]. Favipiravir is a prodrug and is available as a derivative of pyrazine carboxamide [8]. It is used to treat mild to moderate symptoms in adults with a great reduction in viral load, under ‘restricted emergency use’. For a better therapeutic effect, it is used in combinations with other drugs, such as ivermectin, remdesivir, tocilizumab, and hydroxychloroquine [33].
2.1.4.2. Mechanism of Action
Favipiravir works by the formation of a complex with RdRp of the virus [33]. It terminates the RNA chain of the virus by inhibiting the replication of the virus and thus decreases the RNA viral load [37]. The exact mechanism of action of it against SARS-CoV, SARS-CoV-2, and MERS-CoV is still under clinical trials and has passed Phase I and Phase II successfully [33].
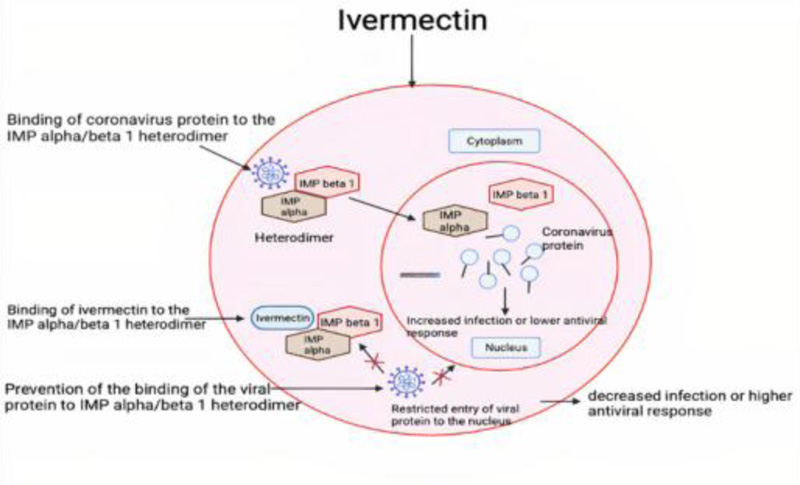
Favipiravir is recognized as a purine nucleotide by the viral RdRp [33, 37]. After penetration, the prodrug is converted into its active metabolite ribonucleoside 5’ triphosphate, inhibiting viral strand replication [2, 6]. It ultimately targets the RdRp enzyme in the virus, which is important for the transcription and replication of the viral genome [38]. It is most effective in viral infections of the lungs, brain, liver, kidney, spleen, and plasma.
2.1.4.3. Administration
Favipiravir is the first oral antiviral approved for the treatment of SARS-CoV-2. It is given for 14 days to the infected patients with the dose of 1800 mg two times a day on day 1 and 800 mg twice daily up to 14 days [33, 38]. It showed a great reduction in viral replication when the dose was adjusted between 700-1400 mg /kg/day [6] and toxicity at higher doses [39]. The pharmacologic effect of favipiravir is more pronounced when it is used in combination with other drugs, such as antimalarial hydroxychloroquine and a cytokine (IL-6) blocking agent [33].
2.1.4.4. Adverse Effects
Very few side effects are observed, like diarrhoea, hyperuricemia, alteration in the blood cell count, and hypersensitivity to other drugs [8, 39]. It should be immediately discontinued if any allergic reaction persists [39]. It is still under clinical investigation for more details about adverse effects.
2.2. Antibiotics
2.2.1. Doxycycline
2.2.1.1. Indications
Doxycycline (DOX) is a broad-spectrum antibiotic that exhibits antiviral as well as immunomodulation effects [40-42]. It is usually given to patients with ocular rosacea and posterior blepharitis [43]. DOX is a drug of choice in the treatment of acne and mycoplasma pneumonia [40, 44]. It is a semi-synthetic derivative of oxytetracycline [45]. It is administered orally in patients suffering from mild to moderate pneumonia [46]. DOX is used in COVID patients to recover the sense of smell [40]. According to the results of the clinical trials conducted by Nandrakumar et al., the recovery rate of patients administered Doxycycline is 85% [47].
2.2.1.2. Mechanism of Action
DOX acts on the IL-6 pathway, IL-1B, and TNF, thereby inhibiting cytokine storms [33]. It also inhibits NLRPs (Nucleotide-binding oligomerization domain, Leucine-rich Repeat, and Pyrin domain containing) inflammasome activation by regulating the IL-1 beta expression [46]. Clinical outcomes prove that the anti-inflammatory process of DOX is achieved by eliminating the activated T cells. Fas/ Fas Ligand (FasL) mediated apoptosis is the mode of action by which it inhibits the Jurkat T lymphocytes' proliferation and their apoptosis [45].
DOX enters the cell by hydrophilic pores through the outer cell membrane and acts as an ionophore, thereby increasing zinc intracellular concentrations and thus suppressing the viral replication [40, 45]. It is also an inhibitor of metalloproteinases (MMPs) and relies on zinc for its action, thus showing anti-angiogenic action [33, 40]. It may directly affect the life cycle of SARS-CoV-2 by interrupting in proteolysis induction of viral non-structural proteins and inhibition of RdRp [48]. It inhibits the protein synthesis, that is, translocation of the viral RNA by binding to specific mRNA. It diminishes the neutrophil chemotaxis and inhibits the viral entry by reducing CD147 expression. When used in combination with hydroxychloroquine (HCQ) and colchicine, it was clinically observed that the drugs decreased the overall mortality rate and improved the rate of recovery [41]. DOX inhibits the protein synthesis by blocking the 30S ribosomes and thus affecting translocation [41].
2.2.1.3. Administration
DOX is given orally once or twice daily to the infected patients [42]. It should be administered with sufficient amounts of fluid and avoided to take it at bedtime (risk of esophageal irritation) and intravenously to avoid the risk of thrombophlebitis [45]. The dose is generally adjusted between 40 mg to 100 mg depending on the condition of the patient [40]. The half-life of the drug is 15-16 hours after one dose and 22 hours after multiple doses [44, 45]. It is contraindicated in patients if they are allergic to it or breastfeeding [48]. It is excreted with urine and faeces [49].
The side effects include headache, nausea, vomiting, and sensitivity to light [42, 46].
2.2.2. Azithromycin
2.2.2.1. Indications
This drug is an antibiotic and belongs to the azalide group [50]. It also shows antiviral effects against the Ebola virus, Zika virus, and Influenza H1N1 virus [51]. It also shows anti-inflammatory and immunomodulatory action against viral RTIs [52]. It is effective in the treatment of cystic fibrosis, non-CF bronchiectasis, chronic rhinosinusitis, and sepsis [53]. It is used to treat COVID patients to prevent bacterial co-infections. AIFA (Italian Drug Agency) recommended that the drug should not be used in COVID-19 patients unless a bacterial superinfection is observed [54].
2.2.2.2. Mechanism of Action
Azithromycin prevents the translation of mRNA by binding itself to the 50S ribosomal subunit, thereby inhibiting protein synthesis and targeting the lysosomes; however, it is ineffective in nucleic acid synthesis [54]. It shows potential synergistic effect when used with other antiviral drugs [55] which is mediated by IFN pathway and reduces replication of the Rhinoviruses [56, 57].
Azithromycin inhibits CRS (cytokine release syndrome) or cytokine storm, thus decreasing the inflammation in the lungs [52]. It influences the mitogen-activated protein kinase (MAPK) and shows the effects on extracellular signal-regulated kinases (ERK ½) and NF-kB [54].
2.2.2.3. Administration
It is generally given orally with an initial dose of 500 mg, which reaches peak plasma concentration at 0.4 mg/L [53]. Its distribution in the body is much higher in tissues than in blood or plasma [57]. It is eliminated by biliary excretion and with urine [56]. The half-life of the drug is 68 hours [55].
It has been observed that azithromycin (500 mg on the first day followed by 250 mg on subsequent days) when given with HCQ (600 mg) for 6-7 days resulted in maximum patient recovery in less time as compared to azithromycin given alone [51, 56]. Azithromycin (2g/day) + Oseltamivir is also beneficial in influenza-like symptoms but not in inflammation [51]. Drug administration is also associated with the most common adverse effects like arrhythmia (QT prolongation), coronary artery disease, polymorphic ventricular tachycardia, and cardiac toxicity [52, 55]. Studies are still going on to understand the exact mechanism and pharmacology of the drug on COVID patients [33].
2.2.3. Amoxicillin
2.2.3.1. Indications
Amoxicillin is a narrow-spectrum antibiotic that is a class of hydrophilic amino penicillin and belongs to the class of beta-lactam antimicrobials [58, 59]. It is used to treat respiratory tract infections, genitourinary tract infections, ear, nose, and throat infections [58, 59]. Though amoxicillin is an antibiotic, it is used to treat patients suffering from SARS-CoV-2 [58]. Its effectiveness has been reported in the bacterial infections that accompany the attack of the virus in the lungs [60]. The exact mechanism is still under clinical research, but proven therapeutic effects are discussed.
2.2.3.2. Mechanism of Action
It is directly integrated into the group NH2 SB-15, which is directly proportional to the pH and the concentration of the solvent used [58, 60]. The release of the drug from the group depends on the physical state of the material. The activity of amoxicillin is related to the inhibition of protein synthesis.
2.2.3.3. Administration
Amoxicillin is well tolerated by the body, though there are some risks associated with the administration of the drug, including GIT infections, toxicity, angioedema, purpura, thrombocytopenia, granulocytopenia, and leukopenia [59]. It also causes some hypersensitivity reactions like Type I, II, III, and IV [60]. It is given orally as an immediate release or extended-release tablet that is taken one hour after the meal, and the dose is adjusted between 125-250 mg depending on the severity of the condition [60, 61]. It is excreted from the body by tubular secretion [61].
2.3. Immunomodulators
2.3.1. Tocilizumab
2.3.1.1. Indications
Tocilizumab (TZ) is a drug of choice that was initially approved for autoimmune disorders like Rheumatoid Arthritis and Systemic Juvenile Idiopathic Arthritis because of its anti-inflammatory property [62]. During SARS-CoV-2, there is an increase in cytokine levels inside the body that initiate CRS, the JAK-STAT, and the pathway of Interleukin 6 (IL6) [63, 64]. In most severe cases of COVID, there is a tremendous increase in cytokine levels known as ‘Cytokine Storm’ [64, 65]. TZ acts as an antagonist to this and is a blocking agent of cytokine (IL 6) [65, 66]. It is also a pro-coagulant. It acts as a human monoclonal antibody and helps in prolonged overall survival, improves oxygenation, and acts as an anti-inflammatory drug [67, 68]. It suppresses fever, lowers C- reactive protein, decreases blood lymphocyte count, increases oxygen intake, and improves CT scan imaging [63]. It reduces the risk of COVID from being a critical disease by eventually helping the patient not to depend on mechanical ventilation [66]. A study by Stone et al. concluded that TZ was not efficacious in hospitalized moderately ill patients, but it worsened the condition of the patients receiving TZ and standard care [63, 64, 66]. On the contrary, a trial conducted by Salama et al. concluded that TZ might help in preventing the condition from worsening by reducing the dependence on mechanical ventilation but did not improve survival [69]. The mortality rate in patients is related to cytokine release, known as CRS [64, 65].
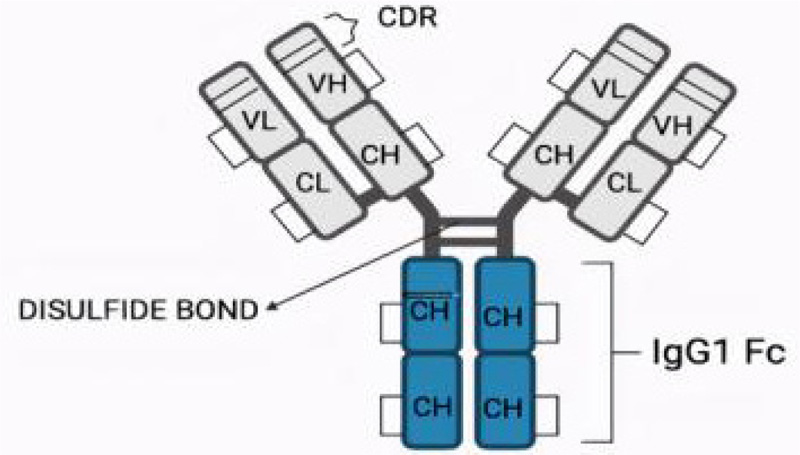
2.3.1.2. Structure
The structure of TZ consists of two heavy chains and 2 light chains with 12 intrachain and 4 interchain di sulphide bonds [67]. Studies are still going on by scientists to understand the complete structure of TZ (Fig. 4).
2.3.1.3. Mechanism of Action
Cytokine storm results in lymphopenia that is responsible for defective T and B cell-dependent immune responses [62]. It also leads to the overexpression of multiple pro-inflammatory cytokines, chemokines, and growth factors, such as IL-6, IL-1 beta, IL-8, IP-10 (Interferon-gamma induced protein), and GM-CSF (Granulocyte-macrophage colony-stimulating factor). It exerts its pharmacological effect by interacting with the IL-6 binding epitope of IL-6R [67]. This improves the oxygenation level inside the lungs but, as such, does not affect the mortality rate [64]. For drug-drug interaction, TZ inhibits the down regulation of the CYP450 enzyme system induced by IL-6 [65]. This will lead to a decrease in the mortality rate in 30 days. During severe lung infection, higher IL-6 levels lead to a hyperinflammatory syndrome known as coagulopathy, which is the major reason for death in COVID-19 patients. It reduces the expression of TNF and IL-10, thus inhibiting monocyte phagocytic activity [63].
2.3.1.4. Administration
It is given intravenously to hospitalized patients suffering from extensive lung lesions. The dose is adjusted between 4 mg/kg to 8 mg/kg depending on the condition and is generally given as a single dose within 14 days of infection [62]. TZ has a nonlinear pharmacokinetic profile, showing an almost constant effect at 800 mg [62]. The long half-life of the drug is 5-12 days. It should be given at an early stage of COVID-19 for beneficial effect [62, 64].
2.3.1.5. Adverse Effects
It also shows severe adverse reactions like nausea, mouth ulcerations, abdominal pain, gastritis, hypertension, headache, and the possibility of secondary infection also [67]. However, it is still under investigation for its mechanism of action in covid patients. It does not decrease the mortality rate in patients but improves the oxygenation level while reducing the dependency of patients on mechanical ventilation [64, 65, 68, 69].
2.4. Anti-inflammatory
2.4.1. Colchicine
2.4.1.1. Indications
Colchicine is a lipid-soluble alkaloid that has anti-inflammatory and antiviral effects [70]. It is a potent inhibitor of NLRP3 inflammasomes, which are primarily responsible for acute respiratory distress syndrome/ acute lung injury (ARDS/ALI) [41]. It is a potent drug used for the treatment of acute pericarditis, gout, and prevention of atrial fibrillation that occurs post-cardiac surgery and post-ablation procedures [71, 72].
2.4.1.2. Mechanism of Action
It works on two levels by inhibiting P2X7 receptor activation and ASC polymerization, thereby blocking the interaction between pyrin-like domains [73-75]. Colchicine also suppresses the transport of mitochondria and the subsequent approximation of ASC to NLRP3 [75]. Moreover, it suppresses the production of IL-1b, IL-18, and IL-16 production [73].
It has an anti-inflammatory effect on neutrophil chemotaxis [72]. By binding to tubulin proteins, it distorts their capability of mitosis, thereby showing anti-inflammatory action [70]. Colchicine also combats SARS-CoV-2’s inflammatory effect on myocytes and shows its effects on the heart also [74].
2.4.1.3. Administration
Colchicine is given orally on the eighth day of infection with a loading dose of 1.5 mg followed by subsequent doses of 0.5 mg two times a day [70]. When absorbed orally, its bioavailability is 25-50% only [76]. Its half-life in a patient with regular renal and hepatic function is 9 hours, whereas it is 24 hours in a patient with predetermined renal failure [70]. The drug reaches the maximum plasma concentration after 1-2 hours of administration, though the maximum anti-inflammatory effect is observed within 24-72 hours of oral administration as it requires time to accumulate in granulocytes and monocytes [75]. It is metabolized by CYP450 in the liver and intestines and excreted with bile and faeces [76]. The main metabolic pathway is deacetylation in the liver.
The administration of colchicine has shown certain side effects, including nausea, diarrhoea, vomiting, and abdominal pain [77, 78]. It should be avoided if hypersensitivity, hepatic failure, pregnancy, or any allergic reaction persists [76].
The substrate of Colchicine is P-gp and CYP3A4. Hence any P-gp inhibitors may result in colchicine toxicity in the body [77]. Clinical trials are still going on to explore the therapeutic effect and mechanism of action of colchicine in humans with SARS-CoV-2 infection.
2.5. Antimalarials
2.5.1. Chloroquine (CQ) and Hydroxychloroquine (HCQ)
2.5.1.1. Indications
CQ and HCQ belong to the first category of drugs working against COVID-19. HCQ is a derivative of CQ [33]. Previously used for rheumatoid arthritis and Systemic Lupus Erythematosus, it is now accepted as an antiretroviral drug used against SARS-CoV-2 [79]. HCQ is a significant drug for the treatment of malaria [80]. Though HCQ is less toxic than CQ, a potential overload of the drug can still lead to poisoning [80, 81]. It is potentially used for covid treatment as an immunomodulator and anti-inflammatory drug as it can reduce the production of cytokines in COVID patients [81]. However, few studies also reported that HCQ and CQ have no clear benefit on the patients' health; rather, they produce more harm than benefit [82]. In the clinical trials conducted by Elsawah et al., a total of 609 patients were taken, out of which, 294 received HCQ and 315 were controls, and the results turned out to be ambiguous and are still a matter of debate [83].
Despite all these results, it is considered an effective drug used in viral load reduction and hence safer than Remdesivir. HCQ is also expected to reduce the risk of mechanical ventilation [79].
2.5.1.2. Mechanism of Action
HCQ is anticipated to show the antiretroviral impact by inhibiting viral glycosylation [81]. It can work by either in-vitro blocking of IL-6 by Toll-like receptor activation that causes inhibition of CD8T response, leading to a decrease in viral load and increase in Interferon production, or by synergistic interaction of IL-6 and IL-T [80-82]. HCQ inhibits the entry of the virus in the cell and post-entry stages, whereas CQ inhibits the entry of the virus by altering the glycosylation of ACE2 receptor and Spike protein [79, 84].
HCQ blocks the transport of SARS-CoV-2 from early endosomes (EEs) to endo lysosomes (ELs), which is required to release the viral genome. It changes the size and number of EEs and ELs [84]. The untreated EEs show normal size, which is less than Els, but when treated with HCQ, abnormally enlarged EEs are observed, many of which are larger than Els [79, 84]. The increase in size and number of ELs is also significantly observed. The mechanism of action of HCQ also involves the interaction of PD1 and PDL-1, which prevents autoimmunity [80]. During viral infection, it alters the immune response, thereby reducing the CD8T cytolytic function [82]. HCQ also elevates the pH, but due to a lack of study and confirmed clinical results, its effect is still not clear on lysosomes [82] (Fig. 5).
2.5.1.3. Administration
HCQ and CQ work more efficiently when given orally because of the high distribution (200-700 times) inside the liver, spleen, kidney, and lungs than in plasma [82]. 500-600 mg per day is considered safe in the patients [82-84]. When given in combination with other drugs, they act synergistically [80, 84]. HCQ given with Azithromycin shows an increased virus load clearance with the loading dose of 400 mg + 550 mg on the first day, with subsequent maintenance doses of 200 mg + 250 mg for the next 4 days [80, 84]. HCQ + Nitazoxanide also shows a better therapeutic response as they both have different mechanisms and sites of action on the virus [33]. This combination therapy is given for approximately 10 days with the loading dose of 200 mg + 500 mg three times on the first day [33, 84, 85]. Studies are still going on to check the exact therapeutic response of this combination therapy. It is also given with Favipiravir for 2-5 days with the loading dose of 3200 mg + 800 mg on the first day and subsequent maintenance doses for the next 4 days, that is, 120 mg + 400 mg [33].
2.5.1.4. Adverse Effects
HCQ administration shows many adverse effects on critically ill patients, such as gastrointestinal symptoms, pruritus, and dermatological changes. Moreover, adverse effects that occur rarely include cardiotoxicity (may include QT prolongation syndrome) and irreversible retinopathy [80, 82, 84]. Results from a few outpatients COVID-19 trials concluded that the administration of HCQ led to mild side effects, whereas rare serious side effects were also observed in some patients [85]. Furthermore, its overdose leads to visual changes, nausea, hypokalaemia, shock, and convulsions [84].
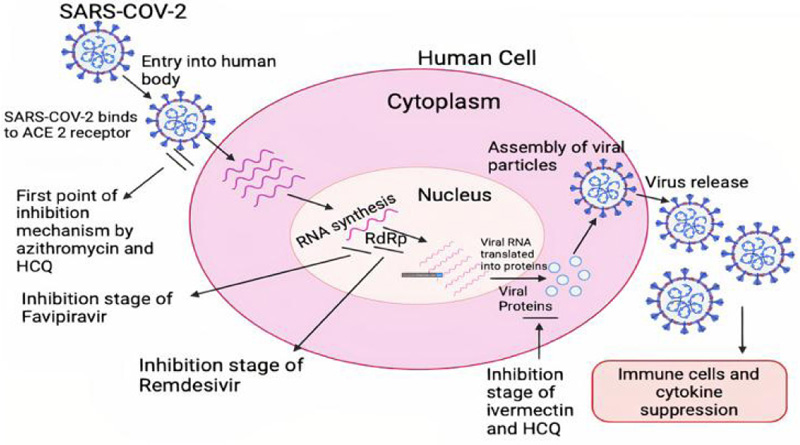
2.6. Steroids
2.6.1. Dexamethasone
2.6.1.1. Indications
Dexamethasone belongs to the class of Corticosteroids, also known as synthetic steroid hormones [86-89]. It acts as a broad-spectrum immuno-suppressive and anti-inflammatory drug used to treat rheumatic problems, allergies, asthma, several skin conditions, brain swelling, and tuberculosis [14, 33, 90, 91]. Steinberg et al. found that corticosteroids, when administered to patients with ARDS, showed an improved oxygen saturation, lowered the mortality rate, and decreased the dependence on mechanical ventilation, thus reducing the risk of death [86, 91]. Dexamethasone can be useful in reducing the inflammation in COVID-19 patients, but it can also hinder the immune responses of the body and hence is still under research [88, 92].
2.6.1.2. Mechanism of Action
Dexamethasone shows an anti-inflammatory effect by 2 mechanisms: Chemotaxis and Vasodilation [91]. It works against COVID by activating the T cells and specific antibodies that inhibit the effects of cytokine storm [87]. The drug, in low doses, follows the genomic mechanism of action and can inhibit the production of pro-inflammatory cytokines, including IL-6, IL-1, IL-2, IL-8, and prostaglandins by a complex formation of the cell membrane with the glucocorticoid receptor (GR) [86, 90, 91, 93]. Whereas, at higher doses, it follows a non-genomic mechanism of action and forms a complex with the membrane-associated GR on cells, especially T lymphocytes [86, 93]. The genomic mode of action is comparatively long-lasting and has fewer side effects compared to the non-genomic mode of action [86].
2.6.1.3. Administration
Dexamethasone is given only for a short period of time, with the dose ranging from 6 mg to 10 mg daily [93]. It is only recommended for hospitalized patients with acute symptoms [90]. In patients with mild symptoms, the drug may be more ineffective than beneficial [86, 94]. It shows several adverse effects on the patient, including hyperglycaemia, increased appetite, mood changes, dizziness, headache, increased sugar level, and agitation [89, 91]. Its intake should not be stopped suddenly, but the dose should be decreased gradually. As the patient leaves the hospital, he must be given Luteolin, which is a potent inhibitor of mast cells and also shows anti-inflammatory and antiviral effects [87]. Clinical research is still going on to study the exact mechanism and pharmacology of the drug when used for SARS-CoV-2 treatment.
2.7. Vitamins and Minerals
2.7.1. Vitamin C
2.7.1.1. Indications
Vitamin C is an antiaging nutrient and effective antioxidant as it elevates oxidative stress and is also used in the prevention of angiocardiopathy [95-97]. It is an important co-factor of various pathways of our immune system [98]. Moreover, it is a water-soluble nutrient responsible for the regulation of the immune system [99]. When given intravenously in critically ill COVID patients, it improves the condition of the patient by reducing dependence on mechanical ventilation but not reducing the mortality rate.
No studies have found that Vitamin C could treat COVID-19 infection [100, 101]. It is basically an immunoregulator and not a therapeutic agent, though it helps in inhibiting cytokine storms [99]. It may reduce the risk of infection, including those associated with human sepsis [100]. It also helps to improve the symptoms of ARDS [96, 97].
2.7.1.2. Mechanism of Action
Vitamin C acts by suppressing inflammation and enhancing immunoregulation. Vitamin C plays an essential role in the metabolism of Co-factors, copper-dependent mono-oxygenase, and iron-dependent dioxygenases [95]. It is clinically recorded that it consistently decreases D- dimer levels and ferritin with a trend of decreasing fraction of inspired oxygen requirements in COVID-19 patients [101]. When given in combination with other antivirals and antimicrobials, the overall improvement may be seen in infected individuals [101]. The mode of action is still unknown, but it is expected that Vitamin C inhibits the activation of nuclear factor kappa – B (NFkB), which is a primary proinflammatory transcription factor [96, 98]. It can also inhibit the production of IL-6 and tumor necrosis factor-alpha (TNF-alpha) and can reduce GM- CSF signaling responses [98, 102, 103]. High doses of Vitamin C, when given intravenously, may reduce inflammation and the chances of sepsis [100].
S.No. | Name of Drug | Results of Clinical Trials |
---|---|---|
1. | Remdesivir | This drug has been approved by FDA to be used in the treatment of SARS-CoV-2, but trials are still going on to know the exact efficacy of the drug [9, 17, 114]. |
2. | Favipiravir | The drug is still under clinical trials and is the first oral antiviral drug approved [33, 38, 114]. |
3. | Ivermectin | Ivermectin is used to improve the conditions of the patients but has not shown satisfactory results [34]. Hence being antiviral, it can be repurposed to be used in the treatment of SARS-CoV-2. |
4. | Chloroquine and Hydroxychloroquine | The results of the studies conducted indicated that the treatment with CQ and HCQ is still under debate and is not proven that it is beneficial, though still used to treat patients [81-83]. |
5. | Doxycycline | Doxycycline is used to improve the symptoms, such as loss of sense of smell [40, 47]. |
6. | Azithromycin | It is an antibacterial drug and is only recommended to be used when there is a severe bacterial infection in COVID patients [50, 54]. |
7. | Lopinavir | The drug does not show beneficial effects in severe COVID-19 patients but may show small benefits in the early stages [25]. |
8. | Amoxicillin | The use of Amoxicillin to treat COVID-19 patients is still under clinical research, and only its therapeutic effects are proven [58, 60]. |
9. | Ascorbic acid / Vit. C | No studies showed that Vitamin C could treat COVID-19 patients [101]. It only helps in reducing inflammation [95]. |
10. | Colchicine | The effect of colchicine in the treatment of COVID-19 is not well-defined, and hence it is used based on its already defined anti-inflammatory property [70, 74]. |
11. | Dexamethasone | The drug is only used to relieve the symptoms and is not an exact known treatment for the disease [91, 92]. |
12. | Tocilizumab | The results of various Clinical Trials state that the drug may help in preventing the condition from worsening by not letting the patients depend on mechanical ventilation, but it does not improve survival [66, 69, 115]. |
13. | Zinc | During viral infections, a deficiency of zinc is observed, and hence zinc supplements are given to overcome this deficiency. Whether it can treat COVID patients or not is still not known [105, 108]. |
14. | Vitamin D3 | Vitamin D3 helps in improving immunity, but its effects on COVID-19 patients are still not known so far [103]. |
2.7.1.3. Administration
Vitamin C can be given orally or intravenously, with the dose adjusted between 50 mg/kg to 200 mg/kg [96]. Its overdose can lead to Acute Tubular Injury (ATI) due to the conversion of ascorbic acid to oxalate in the body [102]. Also, oxalate nephropathy is observed after the overdose within 2 to 8 days of treatment as Vitamin C is degraded to oxalate, carbon dioxide, and L- erythrose in mammals [95, 102].
2.7.2. Zinc
2.7.2.1. Indications
Zinc is a micronutrient known for its anti-inflammatory and anti-oxidant properties [104]. It helps in maintaining the proper function of our immune system, thereby modulating immune responses [105, 106]. Generally, during viral infections, there is a deficiency of zinc in the body of infected individuals, and hence zinc supplements are taken at this time [105]. The immune responses are mediated by this micronutrient as it helps in the maturation of T cells, which occurs in the thymine hormone [104]. Its deficiency in the body, especially in infants and aged people, leads to the failure of the immune system and hence retardation of growth occurs [105]. It is also expected to show antiviral properties and is being widely used for COVID-19 patients, though it is still unclear whether it can treat patients or not [107, 108].
2.7.2.2. Mechanism of Action
There is an increase in cytokine levels in the body during infection, resulting in cytokine storm and inflammation reactions [103]. Zinc works by affecting cytokine production, hence reducing cytokine storms [104]. This reduces the inflammation by inhibiting the activations of NFkB and RdRp, thereby exerting antiviral action [104]. It may also inhibit the protein synthesis in the viral cells at higher concentrations [106]. Its positive effect is observed in infected people, as it improves the immune system [108]. An increased response is observed when given in combination with antimalarials, which is more efficacious as antimalarial drugs are zinc ionophores [107]. It may also be given with other antivirals, though the exact effect is still being studied [106]. Clinical studies are also going on to gather sufficient information regarding the action of zinc on COVID-19 patients [108].
2.7.2.3. Administration
Zinc is administered orally with a dose of 8 mg given to women and 11 mg given to men [107]. It is a safe nutrient, but elevated levels can still cause toxicity, though toxicity is comparatively rare and deficiency is most commonly seen [108]. Intake of higher doses may result in calcium deficiency in the body [105]. In COVID-19 patients, it is generally given in the form of supplements due to deficiency of this micronutrient, leading to loss of taste and smell. In obese people and alcohol addicts, zinc is present in very low amounts and is responsible for weak immune responses [109]. Hence, these individuals are at greater risk when suffering from any viral infection, especially COVID-19.
2.7.3. Vitamin D3
2.7.3.1. Indications
Vitamin D3 shows immuno-modulatory and anti-inflammatory responses [110]. It is responsible for calcium absorption and maintaining proper immune response in the body [111]. The deficiency of this vitamin in the body is responsible for the increased risk of viral infection, especially in children and aged people [112].
2.7.3.2. Mechanism of Action
It is generally given orally to COVID patients, as it decreases the cytokine levels in the body, thereby producing an anti-inflammatory response [113]. However, this effect was observed only in a few and not in all the patients, as the rate of mortality is not greatly affected by the administration of Vitamin D3 [103]. It can also regulate the expression of ACE2 receptors, that is responsible for the entry of SARS-CoV-2 into the cells [110, 111]. Administration of Vitamin D3 during viral infections decreased the risk of ARDS because of the observed deficiency of this vitamin in infected people [113]. Since the deficiency is not observed in all, the exact effect of vitamin D3 in COVID patients is still not clear, as it shows no effect in hospitalized patients or decreases their stay in hospitals [112]. Studies are going on to find the exact outcome of administration of this vitamin in infected patients alone or in combination with other minerals, such as zinc.
CONCLUSION
From this review, it is clear that currently, no drug is being recommended as the potential drug to treat SARS-CoV-2 infection. They are only given to relieve the symptoms after the virus invades the cells. The pharmacology and mechanism of action are still unclear and under clinical trials (Table 2) [114, 115]. It has been found that drugs given in combination with other antiviral and anti-inflammatory drugs, presented a better therapeutic response, when compared to the drug given alone.
The majority of the drug effects were seen in the body as they decreased the viral load and shortened the stay in hospitals. Though they improved the conditions, associated adverse effects should not be avoided, and hence the drugs should be administered with great care, only after the recommendations of the doctor or registered medical practitioner. Further studies are still going on to develop newer and safer drugs that would be more effective against the SARS-CoV-2 virus.
CONSENT FOR PUBLICATION
Not applicable.
FUNDING
None.
CONFLICT OF INTEREST
The authors confirm that this article content has no conflicts of interest.
ACKNOWLEDGEMENTS
Declared none.