All published articles of this journal are available on ScienceDirect.
Prevalence and Impact of Co-infection in Critically Ill COVID-19 Patients
Abstract
Objective:
To determine the epidemiology and impact of co-infection on COVID-19 pneumonia in critical care setting.
Methods:
This was a single center- retrospective study of COVID-19 patients, who were admitted to intensive care unit between March 2020-April 2021. Only patients with microbiological evaluation of blood, respiratory, or urine culture data were included. Community onset bacterial co-infection (COI) was defined as infection diagnosed within 48 hours and hospital acquired bacterial infection (HAI) after 48 hours of hospital admission. Both COI and HAI were further categorized into respiratory, blood or urinary sources. Demographics, laboratory and hospitalization outcomes were compared between patients with and without COI and HAI.
Results:
Among the 141 patients, COI was found in 25/132 patients (19%) and HAI in 41/109 patients (38%). COI was associated with younger age, lower body mass index (BMI) and being from nursing or long- term care facility. HAI was associated with longer length of hospital stay (29 vs 15 days). Staphylococcus aureus pneumonia (31.3%) and Pseudomonas aeruginosa pneumonia (40.0%) were the most common cause of COI and HAI respectively. Overall, gram negative bacteria predominated in both community and hospital acquired pneumonia. Candida was the single most common cause of blood stream infection in HAI (23%). The hospital survival was not significantly different with or without COI.
Conclusion:
In the critical care setting, a significant proportion of patients with COVID-19 had COI and HAI, especially patients from nursing or long-term care facility. Antibiotic coverage for COI and HAI pneumonia should account for the increase in prevalence of Staphylococcus aureus and Pseudomonas aeruginosa respectively.
1. INTRODUCTION
The novel COVID-19 pandemic represents a challenge for the medical community, partly due to limited clinical experience and knowledge gap in potential complications associated with SARS-CoV2 infection. Concomitant bacterial infections or post-viral bacterial infections have been reported in after various viral infections [1-3]. It is estimated that 90% of mortality during the H1N1 pandemic in 1918 was due to bacterial infection [1, 2]. During the H1N1 pandemic no antibiotic was available. This is in contrast to the current wide availability of various classes of antibiotics. Revisiting the prior experience with pandemic associated viral pneumonia, bacterial co-infection increases the mortality and morbidity after viral infection, especially in influenza pneumonia [1, 4, 5]. The pandemic influenza H1N1 of 2009 and the avian influenza of 2013 were both associated with major bacteria co-infection especially of community onset [6-9]. The mechanisms underlying the pathogenesis of post-viral bacterial infections are complex and incompletely understood, but a variety of host and microbial factors have been implicated in these processes, including impaired barrier function, local immune response, bacterial dysbiosis and others [10, 11].
The prevalence and impact of bacteria co-infection in COVID-19 is an ongoing investigation. The reported prevalence of bacterial co-infection in COVID-19 range from 3% - 12% [12-14]. Recent meta-analysis of ICU patients with COVID-19 showed community onset co-infection rate of 15% and the hospital acquired infection rate of 41% [15]. Risk factors for having community onset of bacterial infections include older age, lower BMI, renal disease, admitted from skilled nursing facility, more severe illness and elevated WBC.
The timing of acquisition of concurrent bacterial infection in COVID-19 is crucial as it determines the epidemiology of the type of bacteria, specifically community onset versus hospital acquired infection. Additionally, the presence of mechanical ventilator, length of stay, and monitoring devices such as central lines influence hospital acquired infection.
There is a paucity of data related to the incidence and impact of bacterial co-infection in COVID-19 disease and the effect of adjunct treatment of COVID-19 on bacterial co-infection especially in the critical care setting. The aim of our study is to determine the prevalence and type of organisms in COVID-19 patients admitted to ICU and to be able to guide physicians on the empiric antibiotic therapy of critically ill patients.
2. MATERIALS AND METHODS
This was a retrospective study of patients admitted to our teaching hospital medical intensive care unit (MICU) with COVID-19 infection who underwent microbiological evaluation of sputum, blood or urine between 1st March and 30th April, 2020. All patients who fulfilled these inclusion criteria were sampled. This study has been approved by our Institutional Review Board (IRB #20-04-2064). Informed consent was waived. We only included patients with laboratory confirmed COVID-19 infection (RT-PCR). At that time, all tests were sent out to the Michigan State laboratory approved by the Center for Disease Control and Prevention (CDC). The following patients were excluded: 1) pregnant patients; 2) those aged <18; 3) patients initially admitted for non-COVID-19 reasons; 4) patients who transferred out for higher levels of care; 5) patients who died within 48 hours of admission; 6) treatment limitation placed within 48 hours of admission.
2.1. Case Definitions
The presence of community onset co-infection (COI) was defined as culture positive for pathogenic organism from sputum, blood or urine obtained within 48 hours of admission to hospital. After 48 hours of admission to the hospital, any culture positivity for pathogenic organisms from sputum, blood or urine was categorized as hospital acquired infection (HAI). The bacteria deemed as contaminants were excluded (Supplement Material Table S1). Common organisms contaminant was regarded as true infection if the pathogens were present from different culture sites (blood & urine or blood & sputum) or if deemed as true infection by the treating physician based on chart review. The prevalence of infection was calculated using the number of patients with culture performed (within 48 hours or after 48 hours of admission to hospital) as the denominator divided by the number of patients with positive culture as the numerator.
2.2. Data Collection
The electronic medical record was queried for the patient’s demographics, comorbidities, home medications, corticosteroid administration, procedures underwent, laboratory data (first blood sample obtained in hospital), length of stay and status at discharge. First, we determined whether sputum, blood and urine culture were obtained. Second, we collected information about microbiological speciation. Third, we collected information about the timing of culture collection in relation to hospital admission to determine COI (< 48hours) or HAI (>48hours). All patients were followed until hospital discharge or death.
2.3. Statistical Analysis
Descriptive statistics were expressed as mean ± standard deviation (SD) for numerical data and frequency with percentage for categorical data. Comparison of patient characteristics and acquisition of infection was performed using T-test and chi-square test for numerical and categorical variables respectively. Duration of hospitalization to culture positivity and length of stay were expressed as median and interquartile range to avoid effect of extreme values. Mortality difference between patients with and without co-infection was assessed using Kaplan-Meier survival curves and log-rank test. The date of admission was used as time zero in the survival analysis. Data analysis was performed using SPSS (version 22, IBM, United States) with statistical significance set at p< 0.05.
3. RESULTS
We identified a total of 141 critically ill patients with COVID-19 infection, who underwent microbiological testing for sputum, blood or urine (Fig. 1). Subject characteristics are summarized in Table 1. Their mean age was 63.1±13.3, half were males (53%) and the majority were African-American. Almost one-third of patients were from the nursing or long- term care facilities. Only 9% of the total patients were on immunosuppressive treatment prior to admission. Cardiac co-morbidity and chronic lung disease were present in the 31% and 38% of the patients, respectively. Corticosteroid was administered in 58% of the patients. Among the 141 MICU admissions for COVID-19 infection, 132 had sputum, blood or urine culture performed within 48 hours and 109 had microbiological evaluation after 48 hours of admission. COI was found in 25/132 (19%) of the patients, whereas HAI occurred in 41/109 (38%).
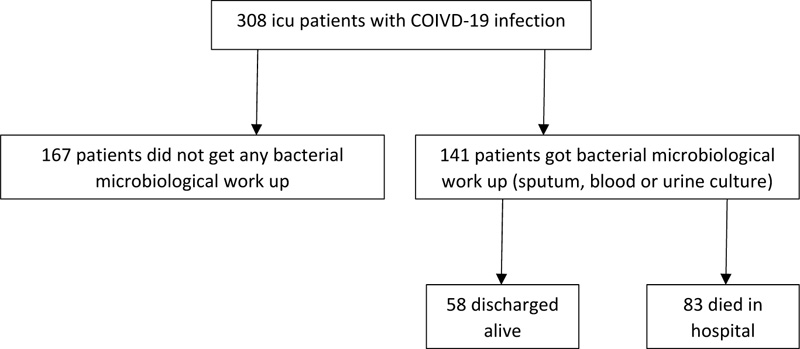
Patients with COI were significantly younger (62.6 vs. 64.3 years) and had a lower body mass index (BMI) (33.2 vs 34.1) compared to those without COI Table 2. Patients with COI, significantly more were from nursing home/long- term care facility as compared to patients without COI (48 vs 13%). White cell counts, lymphocyte and neutrophil counts were not significantly different between patients with and without COI. The rate of COI was not related to the rate of corticosteroid administration. There were no significant differences in co-morbidities between patients with and without COI.
Characteristics | Total, n=141 |
Age, years 20-40 41-60 61-80 >81 |
63.1±13.3 7 (5) 39 (28) 86(61) 9 (6) |
Sex – no. (%) Male |
75 (53) |
Race – no. (%) African-American White Unknown |
109 (77) 2 (2) 30 (21) |
BMI, kg/m2 BMI>40 |
33.7±13.6 32 (23) |
Comorbidities DM HTN Cardiac co-morbiditya Chronic lung diseaseb Chronic kidney disease ESRD Solid organ cancer Hematological malignancy HIV Lung/Renal transplant |
78 (55) 119 (84) 44(31) 53(38) 48 (34) 18 (13) 12(9) 5 (4) 1 (1) 4 (3) |
On immunosuppression prior to admissionc Yes |
9 (6) |
From nursing home, long term care facility Yes |
27 (19) |
Length of stay, daysd ICU |
15(8-25) 11(6-22) |
Alive at discharge Yes |
58 (41) |
Steroid administration after admission Yes |
82 (58) |
Antibiotic given during hospitalization Yes |
137 (97) |
bChroniclung disease included COPD, asthma, interstitial lung disease, sarcoidosis and cystic fibrosis
cImmunosuppressive therapy included corticosteroids, antimetabolite and chemotherapy.
dPresented in median (25th quartile, 75th quartile).
BMI: Body mass index; DM: diabetes mellitus; ESRD: end stage renal failure; HIV: human immunodeficiency virus; HTN: hypertension; ICU: Intensive care unit.
Characteristics | Community onset co-infection | Hospital acquired infection | ||||
Yes n=25 (19%) |
No n=107 (81%) |
P value | Yes n=41 (38%) |
No n=68 (62%) |
P value | |
Age, years | 62.6±3.3 | 64.3±1.1 | 0.02 | 62.4±14.4 | 63.6±11.4 | 0.15 |
Sex – no. (%) Male |
14 (44) |
5 (51) |
0.7 |
23 (56) |
32 (47) |
0.3 |
Race – no. (%) African-American White Unknown |
19 (76) 0 (0) 6 (24) |
84 (78) 2 (2) 21 (20) |
0.7 |
28 (68) 0 (0) 13 (32) |
55 (81) 3 (3) 11 (16) |
0.1 |
BMI, kg/m2 BMI>40 |
33.2±19.0 6 (25) |
34.1±12.1 25 (23) |
0.04 0.8 |
32.6±12.9 9 (22) |
34.3±14.3 16 (24) |
0.7 0.8 |
Comorbidities DM HTN Cardiac/pulmonary co- morbidity Cardiac co-morbiditya Chronic lung diseaseb Chronic kidney disease ESRD Solid organ cancer Hematological malignancy Lung/Renal transplantc |
15 (60) 20 (90) 15 (63) 9 (36) 8 (32) 11 (44) 8 (30) 1 (4) 1 (4) 3 (12) |
59 (55) 92 (86) 56 (52) 35 (33) 42 (39) 35 (32) 10 97) 11 (10) 4 (4) 1 (1) |
0.6 0.4 0.3 0.7 0.5 0.3 0.4 1 0.02 |
22 (54) 34 (83) 20 (49) 14 (34) 8 (20) 16 (39) 7 (17) 1 (2) 2 (5) 3 (7) |
42 (62) 62 (91) 38 (56) 21 (31) 33 (49) 18 (27) 6 (5) 10 (15) 3 (4) 0 (0) |
0.4 0.2 0.5 0.7 0.02 0.2 0.05 1 0.05 |
On immunosuppression Yes |
2 (8) | 7 (7) | 0.8 | 4 (10) | 4 (6) | 0.4 |
From nursing home, long term care facility Yes |
12 (48) | 14 (13) | <0.01 | 14 (34) | 9 (13) | 0.01 |
Procedures performed Tracheostomy placement Hemodialysis Central line, non-tunneled dialysis line |
14 (34) 20 (48) 36 (88) |
5 (7) 27 (39) 47 (67) |
<0.01 0.3 0.02 |
|||
Invasive mechanical ventilationc | 41 (90) | 69 (87) | 0.7 | |||
WBC at presentation ALC ANC |
10.0±5.8 1.17±0.70 7.54±4.80 |
9.8±12.4 1.15±0.94 7.63±9.14 |
0.9 0.7 0.8 |
|||
Length of stay, daysd ICU |
16(9-30) 14(8-30) |
16(8-24) 14(7-27) |
0.6 0.7 |
29(16-44) 27(14-35) |
15(9-21) 11(6-16) |
<0.01 <0.01 |
Duration to culture positive, daysd | 12(9-30) | |||||
Alive at discharge Yes |
13 (52) | 42 (39) | 0.4 | 20 (49) | 24 (35) | 0.2 |
Steroid Yes |
17 (68) | 57 (53) | 0.2 | 32 (78) | 37 (54) | 0.01 |
Antibiotic Yes |
25 (100) | 104 (97) | 0.4 | 41 (100) | 65 (96) | 0.1 |
Pressor Yes |
13 (54) | 63 (58) | 0.7 | 30 (73) | 38 (56) | 0.07 |
bChronic lung disease included COPD, asthma, interstitial lung disease, sarcoidosis and cystic fibrosis
cFisher exact was used because more than 20% of cells had expected count less than 5.
dPresented in median (25th quartile-75th quartile) and Mann-Whitney test used for comparison between groups.
ALC: Absolute lymphocyte count; ANC: Absolute neutrophil count; BMI: Body mass index; DM: diabetes mellitus; ESRD: end stage renal failure; HIV: human immunodeficiency virus; HTN: hypertension; ICU: Intensive care unit.
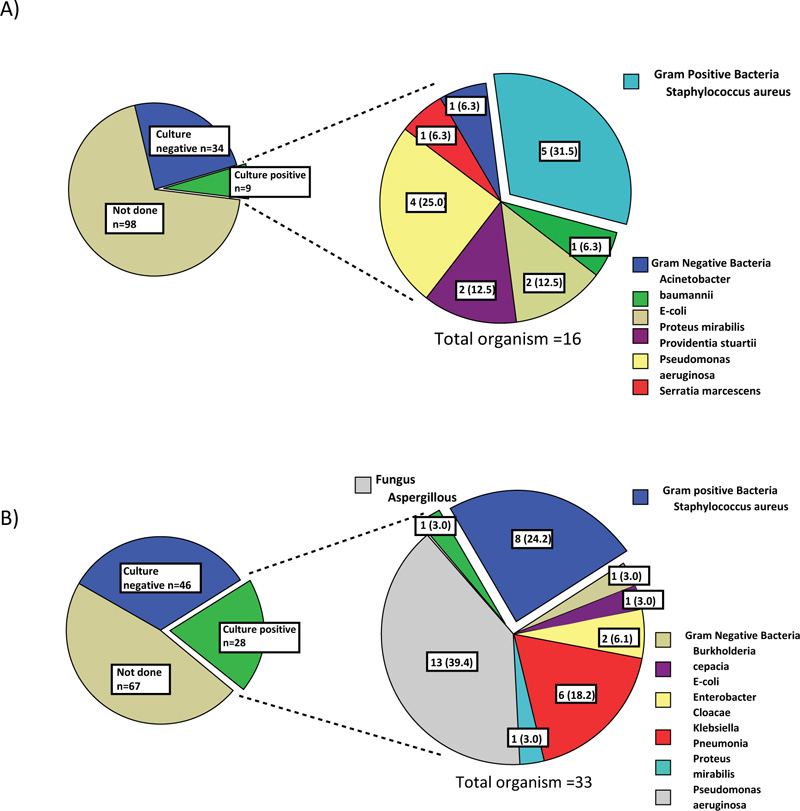
A) 4 patients had more than one organism found on culture
B) 4 patients had more than one organism found on culture
The pie charts on right are presented in n (%).
The most common bacteria found in the sputum culture of the 9 patients with bacterial pneumonia in the COI group was Staphylococcus aureus (31.3%) followed by Pseudomonas aeruginosa (25.0%) (Fig. 2A). It should be noted that six of the nine patients were from nursing home/long-term care facility. Overall, gram-negative bacteria predominated in community-acquired pneumonia. Among 12 patients with COI due to bacteremia, Staphylococcus aureus (23.1%) and Proteus mirabillis (15.4%) were the most common pathogens (Fig. 3A). Overall, COI bacteremia was most likely caused by gram positive bacteria. Urinary tract infection (UTI) in the COI setting was mainly caused by E. coli (40.0%) (Supplement Data Fig. S1).
Patients with and without HAI had similar age (62.4 vs 63.6) and BMI (32.6 vs 34.3). Patients who developed HAI were more likely to be nursing home/long-term care residents (34 vs 13%). HAI was more likely to occur in patients with ESRD (17% vs 5%, p=0.05). In contrast, the risk was not increased in patients requiring invasive mechanical ventilation and it was lower in patients with chronic lung disease (20% vs 49%, p=0.02). HAI was significantly more likely to occur in patients who received corticosteroids (78 vs 54%). HAI occurred on average median (25th quartile - 75th quartile) of 12(9-30) days after the date of admission to the hospital.
Pseudomonas aeruginosa (40.0%) was the most common organism, followed by Staphylococcus aureus (25.0%) as the cause of hospital acquired/ventilator associated pneumonia (Fig. 2B). Aspergillus pneumonia occurred in 3.1% of patients with HAI. Among the 28 patients with HAI pneumonia, 26 were ventilator-associated pneumonia. Similar to COI pneumonia, gram negative bacteria predominated in hospital-acquired and ventilator-associated pneumonia. Candidemia occurred in 23.1% of patients with HAI and this was by far the single most common microorganism found on blood culture (Fig. 3B). Gram negative bacteremia predominated in the HAI setting was mainly due to Pseudomonas aeruginosa (15.4%), Klebsiella pneumoniae (15.4%) and E.coli (7.7%). The causative microorganisms of UTI in the HAI setting were as the following: Candida spp, E. coli, Enterococcus spp, Klebsiella pneumoniae and Pseudomonas aeruginosa.
The median length of hospital stay among our patients was 15 [8-25] days. The presence of COI was not associated with a difference in length of hospital stay. (Fig. 4) illustrates the in- hospital survival curve of patients with follow up duration up to 125 days. In hospital survival was not significantly different between patients with and without COI (p=0.2) (Fig. 4A). About half of these patients were still alive at 25days. On the other hand, the development of HAI was associated with a longer length of hospital stay (29 vs. 15 days, p<0.01). Higher survival rate was associated with the development of HAI (p<0.01) (Fig. 4B). Half of the patients without HAI were alive at 25 days. In contrast, more than three quarters of patients with HAI were still alive at the same duration. Although COI and HAI tended to have a higher rate of being alive at the discharge (52 vs 39%) and (49 vs 35%), respectively, these were not statistically significant (Table 2). Administration of corticosteroid was associated with improved survival (Supplemental Data Fig S2).
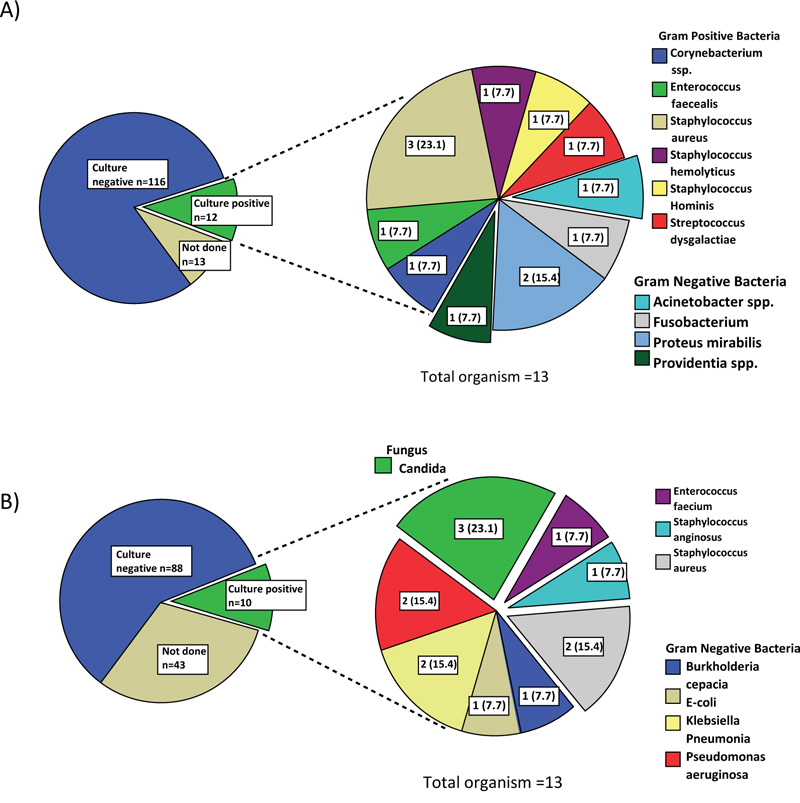
A) 1 patient had more than one organism found on culture
B) 2 patient had more than one organism found on culture
The pie charts on right are presented in n (%).
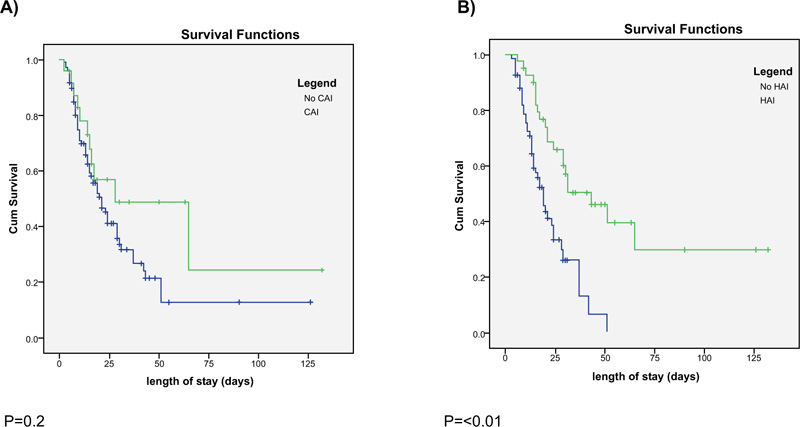
COI: Community onset co-infection, HAI: Hospital acquired infection.
4. DISCUSSION
An earlier publication on bacterial infection in COVID-19 patients from China, as summarized in the meta-analysis by Langford et. al showed 3.5% and 14.3% prevalence of COI and HAI, respectively [16]. The prevalence of bacterial infection can guide antibiotic administration, which is important in the ICU setting as critically ill patients have less reserve for bacterial infection. Our study showed the prevalence of COI at 19% and HAI 38% in patients with COVID-19 requiring ICU care. This infection rate is higher than the reported incidence of COI and HAI (3.1% and 4.7% respectively) by Garcia-Vidalet al. in hospitalized patients with COVID-19 [17]. In our study, we included only critically ill COVID-19 subjects, who would have more likelihood of developing co-infection. This may account for differences in prevalence, as only 12% of Garcia-Vidalet al. cohort were ICU patients.
Several studies reported a higher preponderance of Staphylococcus aureus causing bacterial co-infection in COVID-19 patients. These findings corroborated with our findings [17, 18]. Staphylococcus aureus was also the most commonly identified bacteria in COI associated with influenza pneumonia [8]. Overall, gram negative bacteria predominated in both COI and HAI pneumonia in our cohort. Hence, antibiotic selection should not only focus on Staphylococcus aureus but also should address gram negative bacteria. Although rare, fungal infection had been cited as a cause of pneumonia and blood stream infection in the HAI setting. Hugheset al. reported 3 cases of candidemia and 3 cases of suspected aspergillus pneumonia among 836 patients with COVID-19 [18]. Similarly, Garcia-Vidalet al. reported 7 cases of aspergillus pneumonia and 4 cases of candidemia HAI among 989 patients with COVID-19 [17]. We identified one case of aspergillus pneumonia and 3 cases of candidemia. Our findings were comparable to other studies. Recently, many fatal cases due to mucormycosis in COVID-19 has been reported in India [19]. Hence, depending on clinical suspicion, fungal work up and treatment should be considered.
Several distinct mechanisms contribute to post viral secondary bacterial co-infection: 1) virus-mediated enhancement of bacterial infection; 2) bacterial colonization predisposing viral infection due to downregulation of innate immune response, and 3) hospital acquired infection due to colonization of upper respiratory tracts [11]. Our study distinguishes hypotheses 1), 2) versus 3) based on the timing of diagnosis (48 hours before or after hospital admission) but our retrospective data did not enable us to distinguish between 1) versus 2). Similar to previous studies, our study showed a higher rate of COI in subjects residing in long-term care facility [14]. Since residents of long term care facility have increased bacterial colonization and infection, especially of their respiratory tract [20], hypothesis 2) is more likely to be the predominant cause of COI in our cohort. This can also suggest to clinicians to have a lower threshold for starting antibiotics in these COVID-19 patients.
The pathogenesis of bacterial co-infection after hospitalization is partly influenced by corticosteroids, antibiotic administration, and insertion of lines and endotracheal tubes. COVID-19 associated ARDS leads to prolonged mechanical ventilation with a potential of increased risks of ventilator associated pneumonia. About 8-25% of intubated patients develop ventilator associated pneumonia [21, 22]. According to the meta-analysis performed in the 2016 Infectious Disease Society of America (IDSA) HAP/VAP guideline, the prevalence of bacteria was: methicillin-resistant S. aureus (MRSA) 10%, methicillin-susceptible S. aureus (MSSA) 6%, Pseudomonas species 13%, enteric gram-negative bacilli 16%, and Acinetobacter species 4% [23]. Among the 74 patients with sputum culture, we identified 26 cases of culture-proven ventilator associated pneumonia with bacteria prevalence similar to the above meta-analysis. Critically ill patients often have compromised mucociliary clearance and disrupted mucosal barrier, especially in those with endotracheal tube in place which allows for pathogenic bacterial colonization, especially gram negatives [24-26]. Furthermore, dysbiosis of the upper respiratory tract predisposes nosocomial pneumonia [27].
We observed no increase in in-hospital mortality in the presence of COI. This finding is in contrast to epidemiological data in H1N1 showing COI being an independent risk factor for ICU mortality with OR 1.4 (1.1-1.8, p=0.02)(8). Nonetheless, 97% of our patient cohort received antibiotic, which may have effectively treated the COI and thus narrowing the mortality gap between patients with and without COI. However, patients with HAI had a significantly prolonged length of stay. The better in-hospital survival and longer duration of stay (29 vs 15 days) in our HAI positive cohort are likely because these patients survive long enough to acquire such infection. Although the evidence showed that corticosteroid improves mortality in COVID-19 pneumonia [28, 29], the failure to improve the morbidity of COVID-19, such as prolonged respiratory support and length of hospital stay, which in turn leads to higher HAI [30, 31] should not be overlooked. On the other hand, the development of HAI leads to increase length of stay [32]. The presence of HAI has been reported to add 5 days to the length of hospital stay [33]. This can be the alternate explanation of the association between HAI and prolonged length of stay in our study.
There were several limitations to this study, first, this was a single-center retrospective study. Therefore, microbiological sampling of sputum, blood and urine to evaluate for COI and HAI were not done systematically. The reported infection rate was dependent on whether sputum, blood and urine were ordered by the managing ICU team. Since the majority of patients received empiric antibiotics, cultures may not have been drawn routinely and cultures that were drawn may have been falsely negative. Unmeasured factors such as overwhelming patient loads during the pandemic might have affected the rate of microbiological surveillance as well as the rate and placement of central lines and hemodialysis catheters in suboptimal locations (i.e. femoral) or other monitoring devices during ICU care. Also, the high rate of nursing home/long-term care facility patients may have skewed the data toward specific organisms.
CONCLUSION
In conclusion, in patients admitted to ICU for COVID-19 pneumonia, a significant proportion had concurrent COI and many acquired HAI, especially patients from nursing or long-term care facility. Unlike COI in influenza pneumonia, we did not find that COI in COVID-19 pneumonia causing an increase in mortality. Antibiotic coverage for COI and HAI pneumonia should account for the increase in the prevalence of Staphylococcus aureus and Pseudomonas aeruginosa, respectively.
LIST OF ABBREVIATIONS
BMI | = Body Mass Index |
HAI | = Hospital Acquired Infection |
AUTHORS' CONTRIBUTIONS
Chan Yeu Pu: Conception and design, data acquisition, analysis and interpretation of data. Draft and approval of submitted manuscript.
Morvarid Elahi: Data acquisition and analysis. Draft and approval of submitted manuscript.
James A. Rowley: Conception and design and interpretation of data. Draft and approval of submitted manuscript.
Lobelia Samavati (LS): Conception and design and interpretation of data. Draft and approval of submitted manuscript.
ETHICS APPROVAL AND CONSENT TO PARTICIPATE
This study has been approved by our Institutional Review Board (IRB #20-04-2064).
HUMAN AND ANIMAL RIGHTS
No animals were used in this research. All procedures performed in studies involving human participants were in accordance with the 1975 Declaration of Helsinki, as revised in 2013.
CONSENT FOR PUBLICATIONS
Informed consent was obtained from all participants.
STANDARDS OF REPORTING
STROBE guidelines were followed.
AVAILABILITY OF DATA AND MATERIALS
Data associated with this paper is available under certain circumstances if requested.
FUNDING
The work was partly supported by a grant by NHLBI (R01HL150474) to LS.
CONFLICT OF INTEREST
The authors declare no potential conflict of interest.
ACKNOWLEDGEMENTS
Declared none.
SUPPLEMENTARY MATERIAL
Supplementary material is present on the publisher’s website along with the published article.