All published articles of this journal are available on ScienceDirect.
The Extrapulmonary Manifestations of SARS-CoV-2
Abstract
SARS-Coronavirus 2 (SARS-CoV-2) is the latest strain of coronavirus that causes the viral infection, Severe Acute Respiratory Syndrome (SARS). The initial studies on the Coronavirus Disease 2019 (COVID-19) focused on respiratory outcomes of this viral infection. More recent research on the mechanism of action of SARS-CoV-2 shows that the virus enters the cells through the Angiotensin-Converting Enzyme-2 (ACE-2) receptor. This receptor is present not just in the cell membranes of respiratory cells but also in the cell membranes of cells present in other organs of the body. This enables the virus to have severe outcomes in the body beyond the respiratory system. Providing a possible immunizing agent against coronavirus is a major challenge pertaining to the fact that ongoing pandemic has already taken millions of lives. This paper discusses the extrapulmonary effects of COVID-19, with an emphasis on clinical manifestations, mechanism of action, and special focus to management considerations in each of these cases. The essential therapeutics and treatments proposed for dealing with the COVID-19 infection have also been discussed. While the answer to whether these therapies work, successfully controlling the immunoinflammatory response is still unclear, ongoing trials of multiple drugs for this purpose are an excellent way to ultimately reach a product that works successfully.
1. INTRODUCTION
Coronavirus allegedly started from a wet market in Wuhan, located in Hubei province in China,and spread to more than 188 countries and the viral infection, the SARS-CoV-2 (Severe Acute Respiratory Syndrome Corona Virus-2) has caused a global pandemic termed COVID-19 (Coronavirus Disease 2019), taking more than 1.02 million lives as of October 2, 2020, while the number of active cases has surpassed 34.3 million [1-3]. This global catastrophe started with the proposed zoonotic transmission of coronavirus in the large seafood market in China [4]. This event led to the human-to-human transmission of the virus, infecting more people associated with the said wet market in China, to the point that by January 20, 2020, when the first paper documenting this novel coronavirus was published, the virus had reached more than 800 patients in China and international cases were increasing rapidly [5].
The World Health Organization declared COVID-19 a pandemic on March 11, 2020. In that moment, global cases of the novel coronavirus had surpassed 500,000 [6]. SARS-CoV-2 is a member of the Coronaviridae family, a part of the β-coronavirus genera, same as SARS-CoV (Severe Acute Respiratory Syndrome Coronavirus), the coronavirus that caused the 2002-2003 SARS pandemic and MERS-CoV (Middle East Respiratory Syndrome Coronavirus) that was responsible for the MERS pandemic in 2012 [7]. Common symptoms of COVID-19 include cough, fever, sore throat, loss of taste and smell, leg pain [8], while more severe cases report severe pneumonia (15 percent) and ARDS (Acute Respiratory Distress Syndrome) (5 %) [9]. As respiratory symptoms were the most prevalent in patients infected with COVID-19, initial research on the topic failed to identify the extrapulmonary effects of the infection [10].The reason for this multiple body organs damage by COVID-19 is that the ACE-2 (Angiotensin Converting Enzyme-2) receptor that is used by the virus to enter the body is present in cell membranes of cells not only in lungs, but also in arteries, heart, kidney, and intestines [11].
With more research showing that the viral infection could affect many different body systems, more focus was put on studying the extrapulmonary manifestations of COVID-19,an overview of which is shown in Fig. (1). Even though numerous reports studying the effects of COVID-19 on different body systems have been published, the literature on the topic lacks valuable articles that provide the details of the effects of COVID-19 on the different systems of the human body at once. With the urgency surrounding the study of the virus and the struggle to find a viable treatment, more articles must be published that provide the details of the effects of COVID-19 on multiple organ systems of the body so that researchers can easily access this otherwise wildly dispersed information. This review provides an assortment of the extrapulmonary manifestations of COVID-19. This assortment has been presented after conducting the analysis of the current literature on extrapulmonary manifestations of COVID-19, collecting information about the effect of COVID-19 on different body systems and presenting the information at one place, making it easily accessible for scientific and educational use.
2. IMMUNE SYSTEM
The immune system is the first to face and subsequently deal with the negative effects of any infection or disease, and COVID-19 is no exception; the disturbance in the immune system due to the infection has been found responsible for dire consequences of the infection for the lungs. The SARS-CoV-2 can activate both innate and adaptive immune system, both of which can cause extra trouble when they are responsible for hyperinflammation and impaired immune response, respectively [12]. It is true that a weak immune system can decrease the chances of successfully fighting the viral infection, but an enhanced immune response can lead to terrible consequences for patients too, by causing hyperinflammation, which in turn is responsible for causing multiple organ damage and further complications [13].
2.1. Clinical Symptoms
The first and foremost result of the hyper activated immune system is hyperinflammation, whereby an increase in the inflammatory factors like IL-2, IL-7, monocyte chemoattractant protein 1, tumor necrosis factor alpha-1, among others, results in a cytokine storm or hypercytokinaemia [14, 15]. This hypercytokinaemia can eventually result in hyperferritinaemia, cytopenia, and, more importantly, Acute Respiratory Distress Syndrome (ARDS) and acute coronary syndrome.In addition to being found to be credible predictors of morbidity and mortality, this hyperinflammation has been considered highly harmful in terms of succeeding in controlling the infection effectively [14]. Higher levels of ferritin, and IL-6 [16], greater neutrophil-lymphocyte ratio, lesser number of basophils, eosinophils, a larger number of inflammatory cytokines and biomarkers, also, suppressor T cells, B cells, and NK cells were found in low amounts in more serious cases and non-survivors of COVID-19 [17].
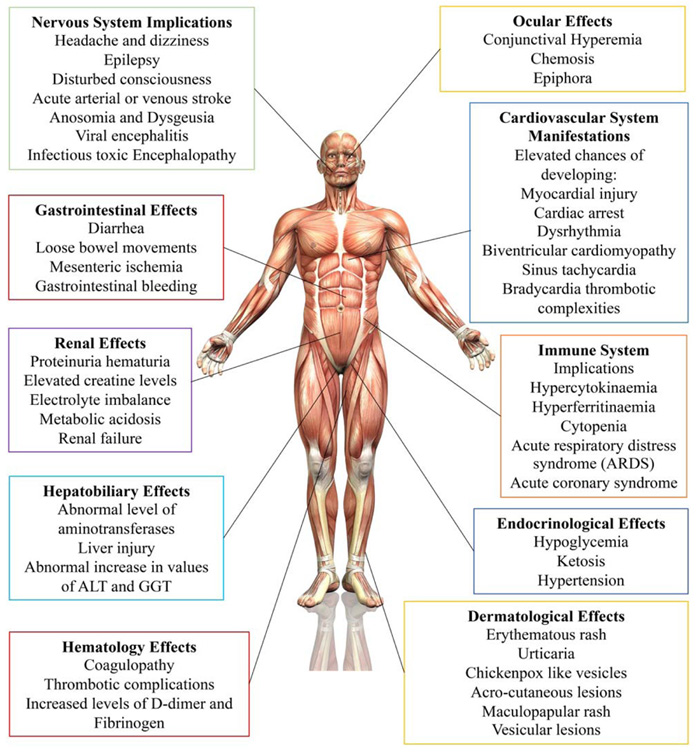
2.2. Pathophysiology
SARS-CoV-2 is one of the cytopathic viruses, and for such viruses, it is often the case that the viral replication cycle itself involves pyroptosis of the virus-infected cells [18] leading to vascular leakage [19], while triggering elevation of IL-1beta [9], an important cytokine involved in the pyroptosis process, ultimately resulting in strong local inflammation due to increased levels of multiple cytokines and chemokines like IL-6, IFNγ, MCP1 and IP-10 [9, 20, 21]. These high levels of chemokines and cytokines attract monocytes and T-lymphocytes from the blood [22, 23], while leaving neutrophils behind, and this movement of immune cells is a viable reason for the lymphopenia and increased neutrophil-lymphocyte ratio seen in most COVID-19 patients [17, 24]. This influx of immune cells, cytokines, and chemokines results in a dysfunctional immune response whereby a cytokine storm is triggered, leading to expansive lung inflammation, and the subsequent difficulty in breathing associated with the infection [25]. In this situation, in addition to the damage being rendered to the lungs by the virus, the unbalanced infiltration of immune cells further damages the lungs by triggering increased secretions of proteases and reactive oxygen species, resulting in alveolar damage, negatively affecting the gas exchange process in the lung, resulting in low oxygen level and difficulty in breathing, making lungs even more prone to secondary infections.The disastrous effect of the infection does not stop here as the increase in the cytokines like TNF results in septic shocks and multi-organ damage, directly resulting in myocardial damage and circulatory failure, decreasing the chances of survival for older patients (aged over 60 years) [16]. However, given that more than fifty percent of child patients and patients under 18 years experienced only mild symptoms, this pattern may not be similar in other patients [26]. That is why, despite the feat of tracking the whole pathway of how COVID-19 results in the pathophysiology of the immune system, there is still a lot left to investigate on how the severity of the inflammatory response in patients is affected by different host immune factors [25].
3. CARDIOVASCULAR IMPLICATIONS
The modest idea of viral infections contributing to the damage to the vital organs has persisted in the scientific community long before the SARS-CoV-2. SARS-CoV-2 affects the cardiovascular system in a way similar to the other members of the ‘beta genus’ family of coronavirus [27]. The clinical implications for COVID-19 patients include moderate to severe damage to the cardiovascular system owing to a particular mechanism [28, 29].
3.1. Clinical Symptoms
The most commonly observed comorbidity of COVID-19 in hospitals is cardiovascular disease (11.9%). Elevated chances of developing a myocardial injury, cardiac arrest, dysrhythmia, biventricular cardiomyopathy, sinus tachycardia, and bradycardia thrombotic complexities along with other cardiac dysfunction and complete heart failure in COVID-19 patients indicate that COVID-19 causes inevitable destruction to cardiovascular health. The experimental study to analyze the cause of death in the coronavirus infected individuals among 150 patients in Wuhan, China revealed that myocardial damage and heart failure were the eminent causes of demise among 53% of patients with confirmed COVID-19 cases among 68 non-surviving patients [16]. The study conducted in 138 patients hospitalized in Wuhan, China, showed that the manifestation of cardiac arrhythmias, heart blockage, ventricular arrhythmias was more prominent in 17% of mild cases and 44% severe cases in ICU [16, 30]. Prolonged QTc (which refers to a slower heart rate indicating increased risk for ventricular arrhythmias) was recorded in 6% of the patients from the cohort of 4,250 patients in New York who were afflicted with the deadly virus. 7–33% of coronavirus infected patients with acute symptoms have been reported to have biventricular cardiomyopathy [16]. Research conducted on 46,248 confirmed COVID-19 patients disclosed that coronary heart disease was present in 1% of surviving and 24% of non-surviving individuals [8].
3.2. Pathophysiology
The mechanism which is involved in causing cardiovascular problems in COVID-19 patients is not clear and well elaborated, but it is proposed that multiple factors are responsible for it [31-39]. SARS-CoV-2 causes severe acute respiratory syndrome as it directly affects the lungs and a complete or partial failure of the respiratory system can produce a strain for the entire body. Hypoxemia results in cardiac stress, which can contribute to indirect myocardial injuries.Immune over-response can also cause cardiovascular diseases, since autopsies of COVID-19 patients show traces of inflammatory infiltrate, which is mainly macrophages [40] and CD4+T cells, the latter are, however, less common. These traces indicate cardiomyocyte necrosis, which could ultimately lead to cardiac arrest [41]. SARS-CoV-2 uses ACE-2 receptors which coat S protein to enter the host’s cell [42]. This receptor is expressed in all types of cells, but their expression is exceptionally more in cardiac cells, which can lead to ACE-2 dependent myocardial infection. Another possible mechanism is the hypoxic state that, in combination with systemic inflammation, increases the production of pro-inflammatory cytokines, which, along with the elevated Ca+2 levels in extracellular space, can result in myocyte apoptosis [13]. To what extent does the heart get affected by a direct viral infection is still unknown. However, the presence and increment of cardiac injury biomarkers highly reflect the possibility of a cardiovascular disorder being a direct and probable cause of SARS-CoV-2 infection in COVID-19 infected people.
4. NEUROLOGIC EFFECTS
The nervous system is another vital organ system of the body that is affected by the SARS-CoV-2, with more than 36.4% of the patient’s cohort to have shown neurologic symptoms [43]. The confirmation for the neurologic involvement of the infection came from Beijing Ditan Hospital, which reported a case of viral encephalitis, with researchers finding SARS-CoV-2 in the cerebrospinal fluid, indicating the attack of the virus into the CNS [44].
4.1. Clinical Symptoms
The extent of neurologic manifestations of COVID-19 is highly dependent on the mildness or severity of the infection itself [24, 45, 46], with mild patients showing symptoms like headache, dizziness, epilepsy, disturbed consciousness [20, 47, 48] and more severe patients showing acute arterial or venous stroke [49, 50], confusion, and troubled consciousness [43, 51]. There have also been recorded numerous instances of COVID-19 patients suffering from anosmia and dysgeusia as numerous reports dictate loss of smell and taste in patients infected with COVID-19 [52]. In addition to viral encephalitis, infectious, toxic encephalopathy has also been reported in COVID-19 patients, mainly due to the hypoxia, viremia, and edema, all of which result due to the infection itself, thereby increasing the chances for the disease [53]. Acute cerebrovascular disease is another neurologic manifestation of the COVID-19, whereby the cytokines storm [14, 54], the reduced platelet levels, and high D-dimer levels caused by the infection are directly related to the factors causing acute cerebrovascular disease [55]. Some reports have also reported the Acute inflammatory demyelinating polyneuropathy (Guillain-Barré syndrome) in COVID-19 patients [56, 57].
4.2. Pathophysiology
Several mechanisms have been proposed for the entry of the virus into the nervous system, based on the previous studies conducted on other coronaviruses like SARS-CoV and some studies conducted on the SARS-CoV-2. These mechanisms include access to the CNS through the nasal mucosa, lamina cribrosa [36], blood circulation pathway [58, 59], olfactory bulb [59, 60], immune injury due to the systemic inflammatory response (SIR) caused by the infection and cytokine storm [54], and attachment of the SARS-CoV-2 to the ACE-2 receptor of the capillary endothelium, damaging the blood-brain barrier and entering the CNS by attacking the vascular system [61-67].
5. GASTROINTESTINAL IMPLICATIONS
Fever, dry cough, and tiredness have been the most associated and most common symptoms of COVID-19. With the prevalence of the disease and increased systematic investigations, it became evident that gastrointestinal symptoms, including diarrhea, nausea, vomiting, and abdominal pain, were also present. This specified the involvement of gastrointestinal disorders and revealed that the digestive system could also be affected by SARS-CoV-2 [68-74].
5.1. Clinical Symptoms
3% of the patients infected with the COVID-19 in Wuhan, admitted to ICU, developed diarrhea [9]. The onset of gastric dysfunction was more notable in patients with intense and more severe symptoms of coronavirus than those who were asymptomatic or showed relatively mild symptoms. The data recruited for approximately 40 days from the three hospitals of Hubei in China revealed that 47% of COVID-19 patients developed digestive system disorders along with respiratory system problems, and 3% developed only digestive dysfunction. Loose bowel movements were recorded in 17%of confirmed coronavirus patients [75]. Rare cases reported mesenteric ischemia along with gastrointestinal bleeding. Severe clinical outcomes are persistent in patients with coronavirus and gastrointestinal disorders. It is also ubiquitous that patients with gastrointestinal disorders developed acute respiratory syndrome; however, the exact mechanism for this phenomenon is a missing link [36]. As the severity of other symptoms increases, the gastrointestinal manifestations also become pronounced in COVID-19 patients.
5.2. Pathophysiology
How the virus SARS-CoV-2 enters the gastrointestinal tract is still under research. Endoscopy reports of six patients showed the presence of SARS-CoV-2 strain throughout the digestive tract, more prominently in the esophagus, stomach, duodenum, and rectum. ACE-2 receptors are present in the epithelial cells lining the upper digestive tract. Not only the ACE-2 receptors, but also the cleavage of S protein, carried out by cellular transmembrane protease serine 2 (TMPRSS2), is a crucial factor for viral attachment and infusion into the host cell. This serine protease is found abundantly in the colon and ileum, suggesting the invasion of the virus into the enterocytes [76, 77]. Another mechanism observed for gastrointestinal involvement is the reduction in the uptake of tryptophan, which leads to decreased peptide levels in gut microbes, ultimately causing inflammation. Cytokine storm or systemic inflammation also affect the gastrointestinal pathway as a comorbidity of multiple organ responses [78].
6. ENDOCRINOLOGICAL MANIFESTATION
The coexistence of endocrinological disorders with COVID-19 is typical in COVID-19 patients. It is not elucidated in the literature that COVID-19 causes endocrinopathies; however, the pervasiveness of more severe symptoms associated with coronavirus is reported. Some of the patients are directly or indirectly susceptible to endocrinopathies.
6.1. Clinical Symptoms
In the United States of America, 49.7% of COVID-19 patients were hypertensive; obesity was recorded in 48.3%, diabetes mellitus was prevalent in 28.3% patients, and cardiovascular disease was common in 27.8% [79-81]. Center for Disease Control and Prevention in the United States reported that diabetes, hypertension, and cardiovascular diseases were common in 78% of COVID-19 patients in ICU. Multiple endocrine glands and organs could be the possible target of SARS-CoV-2, including the pancreas, adrenal glands, bones, testicles, pituitary glands, and thyroid glands. The worst outcomes of the COVID-19 are increasingly evident in people with obesity. Adrenal dysfunction can cause Cushing syndrome. Testicular diseases cause greater mortality in men [82]. Volume depletion in COVID-19 patients suffering from diabetes insipidus can even lead to hospitalization as higher mortality is reported [83].
6.2. Pathophysiology
The effect of coronavirus on the endocrine system is still under debate. Mechanism with which this virus affects the endocrine glands and organ include elevated hypoglycemia and ketosis. The cytokine storm, along with multiple factors, can bind the virus with ACE-2 receptors on the β cells. The pancreas become affected, resulting in abnormal levels of insulin [36]. Metabolic pathways associated with the endocrine system can be targeted directly by the virus. However, multiple organ involvement can produce disastrous effects on metabolic pathways. Altered mechanisms can induce hormonal imbalances.
7. RENAL EFFECTS
The primary target of SARS-CoV-2 is the alveoli present in the lungs, however some of the evidence suggests that coronavirus can also attack and impair the renal functions. In extremely adverse cases, where symptoms of coronavirus are severe, several kidney-associated diseases are reported. It was found that coronavirus can enter the bloodstream and invade other organs, including kidneys. Samples of plasma collected from COVID-19 patients indicate the presence of viral RNA traces, indicating amultiple organ reach of SARS-CoV-2 and kidney is one of them.
7.1. Clinical Symptoms
Clinical diagnostics present an abnormally higher rate of occurrence of kidney disorders. Acute kidney injury is persistent in patients with chronic respiratory system failures. The health data of 5449 COVID-19 patients hospitalized in New York City exhibited that 1,993 cases (36.6%) developed AKI [84-87]. The exceptional irregularities in albumin, proteins, creatine, and blood urea nitrogen levels were observed, indicating kidney damages in confirmed COVID-19 patients. Data for urine analysis and kidney problems retrieved from clinics, laboratories, and hospitals showed 44%and 26.9% of people with COVID-19 had proteinuria hematuria and hematuria, respectively; blood urea nitrogen anomaly was recorded for 14.1% patients. Similarly, 15.5% of patients were observed to have elevated creatine levels [88]. Renal complexities were present among 75.4% of patients in Chinese patients with COVID-19 [89]. Coronavirus is also found to be involved in chronic kidney diseases, electrolyte imbalances, and metabolic acidosis, which can further contribute to renal failure.
7.2. Pathophysiology
More recently in Guangzhou, Zhong’s lab has been reported to be successful in isolating coronavirus from the samples of urine collected from the corona positive cases. This places kidney as a potent organ affected by SARS-CoV-2 [90]. However, there is no elaborated mechanism that dictates direct viral invasion into kidneys that could lead to renal complications. Indirect pathways, including the excessive production of cytokines, multiple organ damages in response to viral attacks, and systemic effects, could be interrelated, resulting in kidney damages. Extracorporeal membrane oxygenation increases the production of cytokines, which may characterize glomerulonephritis, acute kidney injury, and end-stage kidney disease in COVID-19 disease. Renal medullary hypoxia, renal compartment syndrome, and tubular toxicity could be an outcome of multiple organ damage as high peak airway pressure, intra-abdominal hypertension, rhabdomyolysis induced by cytokines in response to SARS-CoV-2 can produce overall stress for the body [91-93]. Kidneys have a 100 times more ACE-2 receptors than the lungs,increasing chances of viral entry into the renal tissues via an ACE-2 dependent mechanism [88].
8. HEPATOBILIARY MANIFESTATION
Severe COVID-19 is also reported to cause hepatobiliary manifestation, but only mild liver abnormalities are present. Abnormal production of alanine aminotransferase and aspartate aminotransferase has also been recorded. The incidence of chronic liver injury or liver damage has been rarely reported. The presence of an underlying or pre-existing hepatobiliary dysfunction has been linked to increase in the fatality of SARS-CoV-2 patients.
8.1. Clinical Symptoms
Case studies of COVID-19 patients logged in Fifth Medical Center of PLS General Hospital in Beijing province of China showed liver co-morbidities in 2-11%. In comparison, 14-53% of the patients had an abnormal level of aminotransferases. 62% of the patients in ICU in Wuhan, China manifested abnormal levels of AST [94]. During the process of hospitalization in Shenzhen, China, 76.3% of confirmed COVID-19 patients had abnormal liver test results, and 21.5% presented liver injury. 90% of patients with reported liver damage had mild COVID-19 symptoms. Out of these, an abnormal increase in values of ALT and GGT was recorded for 24% of patients [95]. The hepatocellular pattern was shared among all the patients with liver damage. The liver damage was commonly reported; however, the consequences were not fatal.
8.2. Pathophysiology
A well-defined mechanism by which SARS-CoV-2 is involved in liver injuries is not available. The impaired hepatic function may be due to the circulation of the coronavirus through the reticular system. Macrophages are abundantly present in the hepatobiliary system, which are involved in the production of the cytokines that result in systemic inflammation [96]. The virus can bind directly to the ACE-2 receptors present on the epithelium since these receptors are intensely present in the liver and it can be subjected to direct damage [97]. Some of the biological drugs like baricitinib, which are believed to produce promising results when used against SARS-CoV-2 for their antiviral properties, can cause the reactivation of any previously present hepatobiliary disorder. Severe oxygen deficiency and respiratory failure can lead to anoxia, which can cause hypoxic hepatitis [98, 99].
9. HEMATOLOGY EFFECTS
The hematopoietic system and hemostasis are essential systems of the body concerned with the regulation of blood flow in the body [8, 24]. Hematology studies report that COVID-19 has considerable effects on the hematopoietic system and hemostasis in the body [20, 100]. Lymphopenia has been reported in multiple cases, with some studies suggesting 67 to 90 percent of patients exhibiting some extent of lymphopenia [9, 66, 101-103].
9.1. Clinical Symptoms
In addition to a decrease in CD4+ T cells [11, 70], CD8+ T cells [20], and leukocytes [18, 104], a marked decrease in thrombocytes has also been reported, which, although mild (present in 5 to 36 percent of patients), is associated with severe patient outcomes [9, 9, 24, 103, 105]. Another important effect of COVID-19 in this context is coagulopathy, which is directly related with increased levels of fibrinogen, one of the procoagulant factors, and D-dimers, the latter of which is associated with more severe outcomes and worse mortality rate in hospitalized patients, 46 percent of whom reported high levels of D-dimer upon admission [8, 20, 24, 47, 106-108]. Levels of D-dimers higher than 1000ng/mL have been reported to be an important risk factor in hospital deaths [8] with non-survivors having more than 500ng/mL levels of D-dimers coupled with prolonged Prothrombin Time (PT) [108]. Finally, thrombotic complications were also associated with about 30 percent of CoVID-19 patients, as reported in China [109] and Netherlands [110]. In comparison, studies conducted in France and Italy reported 17 to 22 percent of critically ill patients with COVID-19 to have shown elevated levels of thromboembolic events, despite receiving prophylactic anticoagulation [111-114].
9.2. Pathophysiology
Multiple mechanisms have been proposed to understand the pathophysiology associated with the hematology manifestations involved in COVID-19 infection. The cytotoxic activity of SARS-COV-2 due to the ACE-2 dependent entry of the virus in lymphocytes [115-117], depletion in lymphocytes mediated by apoptosis [118-120], and inhibition of lymphocyte proliferation due to lactic acid [121] are suggested mechanisms for the lymphopenia associated with COVID-19. On the other hand, leukocytosis, especially neutrophilia and high levels of D-dimers, have been related with hyperinflammatory response to COVID-19 [110, 111]. As for the thrombotic complication, the uncontrolled inflammation, hypoxia, and viral-mediated effects are related to higher instances of thrombotic complications in COVID-19 patients [36]. Endothelialitis, which is associated with irregularly high expression of ACE2 in endothelial cells, also promotes thromboinflammation, resulting in more severe outcomes [115].
10. DERMATOLOGIC EFFECTS
While the dermatologic effects of COVID-19 have only been occasionally reported, still there have been reports about cutaneous findings in COVID-19 patients. Some patients experienced dermatologic effects before the onset of the disease, while others saw dermatologic manifestations before respiratory symptoms appeared or during the infection [36].
10.1. Clinical Symptoms
The most common dermatologic effects reported include erythematous rash, urticaria, chickenpox-like vesicles, acro-cutaneous lesions, maculopapular rash, and vesicular lesions [122, 123]. Also, studies reported chilblain-like lesions present in less severe cases of COVID-19 while patients with more severe infections reported livedoid or necrotic lesions [76].
10.2. Pathophysiology
While the exact mechanism of these dermatologic effects in COVID-19 patients is still unclear, possible mechanisms include SARS-CoV-2 RNA, cytokine-release syndrome, deposition of microthrombi, and vasculitis starting a hypersensitive immune response in these patients, leading to cutaneous and dermatologic effects [41].
11. OCULAR INFECTIONS
More than a decade ago, the world saw a Severe Acute Respiratory Syndrome (SARS) outbreak.The tear samples of a patient from Singapore were found to contain the SARS coronavirus, indicating ocular manifestation of the disease in patients [124]. In 2020, the same situation was reported with Li Wenliang, the person who first reported the coronavirus outbreak and was himself an ophthalmologist and contacted the virus from an asymptomatic glaucoma patient [125].
11.1. Clinical Symptoms
The ocular effects of Covid19 are apparent as, according to one case series, 31.6 % of COVID-19 patients hospitalized in Hubei province, China reported ocular symptoms. Of these 31.6 % of patients with ocular symptoms, the Reverse-Transcriptase Polymerase Chain Reaction (RT-PCR) result of a conjunctival swab of 16.7 % of patients indicated the presence of SARS-CoV-2 [126]. Common ocular symptoms prevalent in COVID-19 patients include conjunctival hyperemia, chemosis, epiphora, or increased secretions [126].
11.2. Pathophysiology
The scarcity of published research on ocular manifestations of COVID-19 has disabled researchers to find the real mechanism of ocular manifestation of COVID-19. Possible mechanisms of action include i) ocular tissues being directly inoculated by respiratory droplets of aerosolized viral particles, ii) nasopharynx migration via the nasolacrimal duct or iii) lacrimal gland involvement in the hematogenous spread [127].
12. MANAGEMENT CONSIDERATIONS FOR MULTIPLE ORGAN DAMAGE
The immunomodulated damage in COVID-19 is a leading cause of the increasing severity of infections for many patients. Several different immunosuppressive therapies have been initiated for controlling this damage. Trials of corticosteroids [27], IL-6 antagonists, tocilizumab [28], sarilumab, Granulocyte-Macrophage Colony-Stimulating Factor (GM-CSF) targeting drugs gimsilumab [29], lenzilumab [30], and namilumab [31] have been initiated. Novel therapies like cytosorb, which involves absorbing large amounts of cytokines [32]; or Thalidomide, an immunomodulatory agent, is also being employed [33]. Since the fatality rate in patients with an underlying cardiovascular problem is significantly more and the death rate becomes even more critical if the myocardial dysfunction occurs after SARS-CoV-2 infestation, it becomes indispensable to treat such patients with utmost medical attention. Even though there should be made specific considerations for the management of such patients, using Angiotensin-Converting Enzyme inhibitors (ACEi) and Angiotensin Receptor Blockers (ARB) is still questionable.
There is no proof that the use of these therapeutics should be stopped after the viral infection happens. The interaction of cardiac drugs with the drugs administered for COVID-19 can have a potentially adverse effect [72]. Management of dosage should be observed along with encouraging proper diagnostics and prohibiting the random testing of biomarkers for cardiac injury. The recently used drugs for COVID-19; azathioprine, when used in combination with antiparasitic drugs, can result in prolonged QT interval, so precautions must be employed to avoid problem [73]. While the existing literature lacks a detailed course of action for the minimization of the neurologic manifestations of COVID-19, several different guidelines can be adhered to for effective results. These include adhering to the guidelines regarding the ischemic stroke and ensuring the availability of thrombolysis and thrombectomy [62], evaluation of patients with remote video monitoring [36], and using immunomodulatory therapies [63].
Corticosteroids are recently being used as effective antiviral drugs that are easy to administer and economically viable. Precisely monitored use of the drug should be made, and pre-testing for the virus must be ensured to prevent the fatal consequence. An elderly COVID-19 patient with severe ulcerative colitis, treated with corticosteroids, expired [79]. The transmission of the SARS-CoV-2 is mysterious. Since 50% of the fecal material and stool samples of COVID-19 patients show the viral presence so these can be a source of transmission [80]. Separate washrooms should be used to avoid such transmission. Biopsies, endoscopy, and autopsies could be performed to precisely elaborate that lesions from the intestine are produced after the systemic inflammation, or they are the consequence of direct primary infection of the gastrointestinal tract. Early detection and diagnosis lead to early treatment. Continuous detection of pro-inflammatory cytokines and limiting their production by monoclonal antibodies could be helpful for safe treatment [78].
The use of glucocorticosteroids is linked with immunosuppression activity, so their use must be regulated as a compromised immune system can result in more susceptibility to the virus and death if the viral infection has already occurred [84]. Adrenal insufficiency and crisis should be managed, and preliminary medical help should be provided without delay [85]. Endocrinologists must effectively cooperate with other medical specialists to perform unavoidable thyroid nodules surgeries for critical COVID-19 patients [23]. Routine care, precise attention to desmopressin dose, and fluids can be helpful to prevent hypernatraemic dehydration in diabetes insipidus [83]. Since no proper medication is available, special consideration should be made if kidney failure, acute kidney injury, and other renal disorders develop, as it could result in an increased mortality rate among COVID-19 patients. A controversy regarding delaying Renal Replacement Therapies (RRT) is that it could be replaced with the use of loop diuretics to prevent volume overload. If RRT is unavoidable, then the patient’s age, the severity of symptoms of COVID-19 should be observed and monitored to ensure timely and safe replacement of organs.
Hospital staff, Radiologists, and nephrologists should work in collaboration to securely reduce the hemodialysis session for kidney failure [91]. Developing COVID-19 from organ donation is very rarely reported. Still, the kidney has the highest vulnerability for SARS-CoV-2. Special clinical screening and management should be practiced to ensure risk-free renal donation [93]. Due to the scarcity of data concerning the impact of drugs affecting the hepatobiliary system, it is mandatory to observe the biochemical monitoring of the liver [98]. Special attention should be given to the elderly COVID-19 patients who have a long-term history of liver injury since it can contribute to the severity of the disease.
No direct evidence is available that proved the dysfunction of the liver as lethal and deadly in SARS-CoV-2 infection [97]. The effect of drugs on liver damage and hepatotoxicity should be considered in drug designing procedure. Less chronic liver disease diagnosis during the pandemic disables the revelation of the association between liver injury and COVID-19 [99]. While there are no fully approved strategies to alleviate the hematology effects of COVID-19, there are indeed some proposed actions that have been shown to have a positive impact. These include continuous evaluations of blood count, D-dimers, lymphocytes, and fibrinogen in hospitalized patients [35], risk assessments for thromboembolism at repeated intervals, therapeutic anticoagulation [36], and mesenchymal cells to be used for their immunomodulatory, anti-inflammatory, and anti-fibrotic properties [122], in addition to their ability to mediate inflammation around endothelial cells, hence not only reducing inflammation but also checking endothelial dysfunction [42].
Even though the dermatologic effects are not as prevalent, it is important to consider patients’ dermatologic effects before administering any COVID-19 drug like Remdesivir, tocilizumab, or starting any possible treatments for COVID-19 [38]. Such patients should also be inspected on a case-to-case basis to decide the continuation of any biologic therapy for the infection.Lack of research in this field has made it challenging to manage the ocular manifestations of COVID-19. While health care providers are guided to make use of strict care to keep their eyes covered and use protective gear, ophthalmologists have been directed to limit their practice to emergencies and urgent cases as the possibility or mechanisms of transmission of the disease through ocular mechanisms are still unclear [125].
13. COVID-19 THERAPEUTICS, CYTOKINE MANAGEMENT AND DRUG REPURPOSING
It has been established that the SARS-CoV-2 enters the cells through the ACE-2 receptor [128]. It has also been reported that the ACE-2 receptor is expressed more in the lungs [129], showing expression in multiple types of epithelial cells in the airway, for example, the alveolar epithelial type II cells in the lung parenchyma [130, 131]. This over-expression of the ACE-2 receptor is directly related to the severity of the COVID-19 infection [132]. One of the reasons that the over-expression of ACE-2 in increasing the severity of the viral infection is the ability of the ACE-2 receptor to increase the pro-inflammatory cytokines (PICs) in the lungs of infected patients, which has been associated with severe outcomes of the viral infection in the lungs of the patients [133, 134]. Continuous increase in the concentration of PICs in lungs gives rise to a sort of cytokine stormor Cytokine Storm Syndrome (CSS) that includes severe pneumonia in patients, drastically increasing the severity of the infection, also associated with increased mortality [14].
The CSS is defined as a cytokine-mediated hyper-inflammatory response in which the immune system of the host is uncontrollably activated and amplified, resulting in excessive release of cytokines such as Tumor Necrosis Factor (TNF), TNF- α, interleukin (IL)-1 β, IL-2, IL6, IL7 IL-18, IL-9, IL-10, interferons (IFNs), IFN-c, granulocyte Colony-Stimulating Factor (G-CSF), granulocyte-macrophage colony-stimulating factor, fibroblast growth factor, and macrophage inflammatory protein 1 [9, 135-137]. This excessive release of cytokines results in respiratory failure by increasing vascular permeability, leading to the entrance of a large amount of blood cells and fluid in the alveoli, critically damaging the host cells [138]. These cytokine reactions further result in the direct or indirect activation of the coagulation pathway [139].Anaphylatoxins such as C3a and C5a are generated by the complement cascade,whose binding to the complement receptors results in further release of histamine, leukotrienes, and prostaglandins [140]. It is the C5a that induces mast cells, neutrophils, and monocytes to release proinflammatory cytokines such as IL-12, TNF-α, and macrophage inflammatory proteins-1α while also stimulating T and B cells to release cytokines such as TNF-α, IL-1β, IL-6, and IL-8., resulting in the CSS [139].
With studies reporting the role of the CSS in causing hyper-inflammation that often results in the severity of the infection and increased mortality rate [141], more focus is being put in finding drugs that target these cytokines, reducing the hyperinflammation associated with increased severity and mortality of the viral infection. In this regard, many different antiviral, anti-rheumatic, anti-inflammatory, antineoplastic, and antiparasitic drugs are being repurposed and investigated for their positive, immune-modulating effects in severe COVID-19 cases.The antiviral drugs that have been used for COVID-19 patients include atazanavir [142], favipiravir [143], IFN-α2b (interferon) [144], lopinavir-ritonavir [145], remdesivir [146], ribavirin [147], and umifenovir [148]. A study reported significant improvement of clinical symptoms of more than 68 percent of patients subjected to a 10-day course of Remdesivir [146]. Remdesivir was approved by the FDA on May 1, 2020, under an Emergency Use Authorization (EUA) for patients with severe COVID-19 symptoms.Anti-rheumatic drugs have also been used in this regard, with the primary mechanism behind their use being inflammatory cascade inhibition and TNF-α [149], IL-6 receptors, or IL-1Ra blocking [150]. The drugs that have been used include recombinant IL-1Ra antagonist Anakinra [151] and antiarthritic agents, including Baricitinib [152], Etanercept [153], and Infliximab [154]. Another important drug is Tocilizumab [155], an IL-6 signal pathway inhibiting monoclonal antibody (mAb). Numerous reports have suggested that the IL-6 cytokine plays the most important role in the pathogenesis of COVID-19, significantly affecting the severity of the viral infection in the lungs [156].
Approach | Mechanism |
---|---|
Stem cell-based therapeutics | These mesenchymal cells are delivered intravenously where they protect the alveolar epithelial cells present in the lungs and recruit the cells that help prevent pulmonary infection and manage the lung dysfunction [74]. |
Use of Opioids and cannabinoids | These drugs are immune-modulatory, and hence they can enhance the immune function of the body by increasing the immune cell influx, increased production of cytokines and chemokines, and affect viral replication and viral pathogenesis [71]. |
Prophylactic and immune-mediated approaches | The understanding of the viral mechanism of action is unavoidable to use such approaches. Two theories are prevalent in this regard; one is the use of immune suppressors to overcome the hyper inflammation caused by viral load, and the other one accounts for the use of immune potentiators to activate the adaptive immune responses in the host [68]. |
Antiviral agents | These antiviral drugs and vaccines can stimulate immune responses. These drugs can bind to the receptor and block viral entry. The drugs can be protease inhibitors or prevent viral replication by inhibiting viral RNA polymerases [65]. |
Some anti-inflammatory drugs have also been repurposed and are being used to treat COVID-19, including non-steroidal, anti-inflammatory Indomethacin [157], immunomodulatory, and anti-angiogenic agent Thalidomide [158], and steroidal anti-inflammatory drugs like corticosteroids, one of which, dexamethasone, has now been licensed [159]. Some antineoplastic drugs, previously being used in leukemia, are also being tested and repurposed, making them exciting choices for COVID-19 treatment [128]. These drugs include Ruxolitinib, a Janus Kinase (JAK) inhibitor [160] and Ibrutinib, a Bruton’s tyrosine kinase (BTK) inhibitor [34], both of which have been shown to be promising in treating COVID-19 patients. Several ongoing trials are searching for a viable solution to the hyper-inflammation that results due to the COVID-19 infection and causes extensive, multi-organ damage and drug repurposing is a significant part of this research [128]. Hopefully, with more research and investigation, scientists would be able to find more drugs that are better able to deal with adverse effects of COVID-19 on the body. Table 1 summarizes the most common therapeutics being used and tested for COVID-19 for a better understanding of the wide range of treatments being tested to treat this viral infection.
CONCLUSION
While extrapulmonary effects of COVID-19 are being appreciated and more research is being undertaken on the effects of the viral infection on different body organs and organs systems, there is still a need to undertake intensive research into the exact mechanisms that the viral particles use for entering the extra-respiratory tissues in the body, the viral features that make this process easier, and long term implications of these extrapulmonary manifestations of COVID-19. This is important as older adults are the most affected by the viral infection and studying and understanding the multi-organ response to COVID-19 is critical to ensure adequate medical care to COVID-19 patients who survive the infection. In the case of such multi-organ infections, ensuring the survival of patients goes beyond fighting the infection and is more concerned with dealing with the long-term implications of that infection on the body. One way this can be done more effectively is to put more focus on regional and international collaborations to work collectively to understand this poorly understood disease, putting an end to this dreadful pandemic.
CONSENT FOR PUBLICATION
Not applicable.
FUNDING
None.
CONFLICT OF INTEREST
1. None of the authors of this paper have a financial or personal relationship with other people or organizations that could inappropriately influence or bias the content of the paper.
2. It is to specifically state that “No competing interests are at stake and there is No Conflict of Interest” with other people or organizations that could inappropriately influence or bias the content of the paper.
ACKNOWLEDGEMENTS
Declared none.