All published articles of this journal are available on ScienceDirect.
Investigation of in Hospital COVID-19 Mortality and One-year Follow-up of Lung Function and Health Status
Abstract
Background:
Globally, thousands of patients suffer from postacute COVID-19 syndrome, a condition that already affects our health system. Although there is a growing literature upon the long-term effects of SARS-CoV-2 infection, there are up to date only a few reports on long-term follow-up of pulmonary function after severe COVID-19.
Objective:
To investigate risk factors for in-hospital COVID-19 fatalities and to assess the lung function and health status at one year after hospital discharge.
Methods:
Patients who were admitted to the hospital with confirmed COVID-19 and required supplementary oxygen delivery were included in this observational cohort study. Baseline and demographic data and information about hospital stay were obtained by medical charts. Patients were divided in 3 groups: group 1 (intensive care unit (ICU)-invasive mechanical ventilation (IMV), group 2 (high-flow nasal cannula (HFNC) and/or none-invasive ventilation (NIV) and group 3 (regular oxygen delivery treatment). All patients were required to answer health questionnaires at one year after acute infection, while patients in groups 1 and 2 performed dynamic spirometry.
Results:
The study population consisted of 130 patients. Forty five (35%) patients died at the hospital. Risk factors for in-hospital mortality were age, hypertension, ischemic heart disease, and renal disease. We did not find any significant difference in health scales between the 3 groups. Mean values of both FEV1% and FVC% in groups 1 and 2 were detected within the lower normal limits with no difference between the two groups.
Conclusion:
The main result of the study is lung function in the lower limit of normal evaluated at one-year follow-up. There were no significant differences related to initial disease severity in lung function and long-term health status, which suggests that more generous lung function testing even in less severely affected patients, could be indicated.
1. INTRODUCTION
Coronavirus disease 2019 (COVID-19) is caused by a novel coronavirus known as severe acute respiratory syndrome coronavirus 2 (SARS-CoV-2) and has been an ongoing pandemic since spring 2020 [1]. The virus's genetic variation and frequent mutations are a worrying threat impacting the whole world as the virus continues to spread globally [2]. Although the infection's acute phase has been thoroughly explored, the long-term medical consequences and prognosis remain largely unclear [3].
Previous coronavirus epidemics (Severe Acute Respiratory Syndrome (SARS) and Middle East Respiratory Syndrome (MERS)) unfortunately had relatively few and short follow-up studies. They were characterized by a much more lethal acute phase than COVID-19 and significant long-term consequences, including chronic fatigue, psychiatric sequelae, and reduced lung function [4-7].
Globally, thousands of patients report suffering from long-term COVID-19 sequela, often referred to as post-acute COVID-19 syndrome [8]. However, exactly what encompasses this syndrome and how it is best treated is not well defined [3]. There is a concern for permanent lung damage, which is not uncommon for advanced viral pneumonitis [9]. Six-month data from China suggest that the majority of in-hospital treated patients still had not only residual symptoms at follow-up, mostly fatigue, muscle weakness, sleeping difficulties, and anxiety or depression, but also residual lung parenchymal opacities and reduced lung function [10]. These findings have caused increased concern for permanent pulmonary damage. Reports indicate reduced dyspnoea over time and a successive improvement of lung function measured with pulmonary function tests (PFTs) and radiological features [11]. Others report chronic impairment with less or no improvement over time [12].
With this study, we aimed to investigate patients with COVID-19 who were hospitalized during the first pandemic wave, by exploring risk factors for in-hospital mortality and by assessing their lung function and health status one year after hospital discharge.
2. MATERIALS AND METHODS
2.1. Study Design and Participants
This is an observational cohort study in which we identified all patients in the Norrköping region (with a catchment area of approximately 180 000 people) admitted to in-hospital care with laboratory-confirmed COVID-19 during the first pandemic wave (Mars 2020-June 2020) and required some form of supplementary oxygen delivery. Patients were identified by a synoptic journal review of relevant wards focusing on positive COVID-19 test results, oxygen administration, and diagnosis code used in the electronic health record. All patients were invited to answer questionnaires one year after acute infection with COVID-19. Patients were stratified into three groups depending on the need for oxygen delivery as follows: Invasive mechanical ventilation (IMV)/Intensive care unit (ICU) (group 1), noninvasive ventilation (NIV)/high-flow nasal cannula (HFNC) (group 2) or regular oxygen therapy (group 3). Those that had received IMV, NIV, and/or HFNC (patient groups 1 and 2) also performed PFTs with dynamic spirometry following the American Thoracic Society (ATS) guidelines one year after infection [13].
No patients were actively excluded. Out of 204 eligible patients,74 could not participate due to various issues (language issues, severe illness, living in another geographical location, did not respond or withdrew consent). 130 patients were ultimately included for follow-up (Fig. 1). The Swedish Ethical Review Authority granted ethical approval for this study (registration number: 2020-03531). Patients were recruited by telephone or mail. Written informed consents were obtained from all those who were alive.
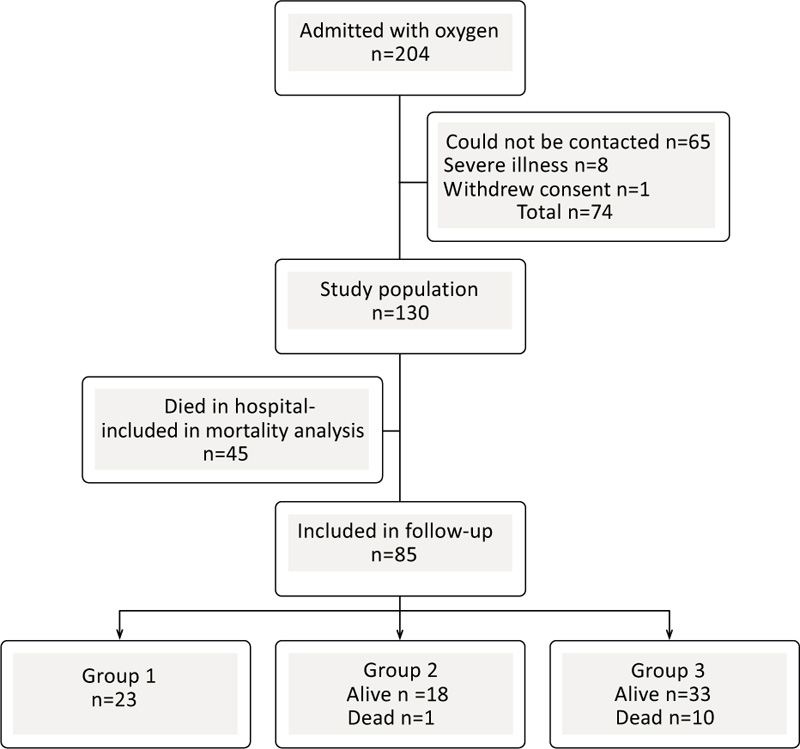
Baseline characteristics, including blood samples, were obtained by manual review of the medical records. Questionnaires were mailed to the patients at the 1-year follow-up, and if they were not completed and resubmitted, patients were contacted by telephone to give supplemental information. The same physicians performed all PFTs with the same level of enthusiasm. The CareFusion MicroLab Mk8 Desktop Spirometer was used. After 1 year, a new review of the medical records was done to collect information concerning mortality, readmissions, unscheduled medical appointments, and radiological examinations. All except one patient in the IMV/ICU-group 1 provided complete information for analysis; however, this patient could not perform spirometry or answer questionnaires related to underlying comorbidity. Based on the radiological report, and patients with available thoracic imaging by computed tomography one year after infection were evaluated for signs of fibrotic pulmonary disease.
2.2. Questionnaires
The modified Medical Research Council (mMRC)Dyspnea Scale is a well-validated scale for the assessment of dyspnoea [14].
Post-COVID-19 Functional Status Scale (PCFS) is a new and validated scale that compares self-reported functional status before and after COVID-19 infection [15].
The Hospital Anxiety and Depression scale (HAD) is a validated screening instrument for depression and anxiety [16].
36-Item Short-Form Health Survey (SF-36) is a broad screening instrument that measures general health in several domains [17].
2.3. Statistical Method
The R-language was used for all calculations and visualisations [18]. Continuous variables were summarized as mean± standard deviation (SD) if normally distributed and as median interquartile range (IQR) if non-normally distributed. Categorical variables were summarized as n (%).
Continuous variables were assessed for normality by use of the Anderson-Darling test for normality [19].
For continuous variables, differences between the two groups were tested for statistical significance with the parametric t-test for normally distributed variables and the nonparametric Wilcoxon test for nonnormally distributed variables.
Differences in distributions of categorical variables across two or more groups were tested for statistical significance with the parametric Fisher Exact Test.
Analysis of variance (ANOVA) was used to detect differences in numerical variables between the three groups.
Associations between continuous variables were tested for statistical significance with regression analysis, although nonsignificant results are not shown.
3. RESULTS
The study population consisted of 130 patients. Their demographic and clinical characteristics at the time of diagnosis of SARS-CoV-2 infection are given in Table 1. Forty-five (35%) patients died in the hospital (non-survivors), while 85 (65%) were discharged (hospital survivors). Out of 34 patients in group 1(ICU/IMV), 23 (67%) patients survived and were discharged. In group 2 (HFNC/NIV) and group 3 (oxygen treatment), 19 (51%) patients and 43 (73%) patients, respectively, were discharged (Fig. 1). Of those discharged, all patients from group 1 were alive one year after infection, while from groups 2 and 3, 1 patient and 10 patients had died, respectively. The median time between hospital discharge and death was 25 (56) days (median (IQR)). Only 2 patients from group 1 had unplanned revisits to the hospital regardless of the reason for admission, while 4 patients from group 2 and 11 patients from group 3 had unscheduled hospital visits within one year after infection. The total hospitalization duration in days was significantly longer for group 1 (34±30 days, mean±SD, p<0.001) than for group 2 (9.6±5.3 days) and group 3 (7.5±5 days).
Age, year (median (IQR)) | 71 (20.5) |
Sex (M/F) | (83/47) |
Alive/Dead (hospital mortality) | 85/45 |
Smoking history, n (%) No smokers Ex-smokers Current smokers |
57(44%) 64(49%) 9 (7%) |
Hypertension | 89(68%) |
Diabetes | 44(34%) |
Heart disease | 58(44%) |
Ischemic heart disease | 39(30%) |
Chronic Obstructive Pulmonary Disease (COPD) | 22(17%) |
Asthma | 13(10%) |
Immunosuppressive treatment | 19(14%) |
Renal disease | 14(11%) |
Neurological disease (includes stroke) | 30(23%) |
Rheumatological disease | 9 (7%) |
Cancer (active and treated) | 15 (11%) |
BMI (n=123) mean± SD Normal weight (n %) Overweight (n %) Obesity (n %) |
29±5 25(20%) 45(37%) 53(43%) |
Symptoms and laboratory data at presentation | n (%) |
Fever | 114(87%) |
Cough | 84 (64%) |
Dyspnea | 98 (75%) |
Gastroenterological symptoms | 22 (17%) |
Laboratory data | |
Lymphocytopenia, n (%) | 61(47%) |
CRP (mg/L) (median (IQR)) | 91 (134) |
Haemoglobin (g/L)(median (IQR)) | 137 (25) |
Troponin (ng/l)(n=127) (median (IQR)) | 20 (32.5) |
Creatinine (µmol/L)(median (IQR)) | 93 (52) |
eGFR (mL/min/1,73 kvm) (median (IQR)) | 66 (42) |
Ferritin (µg/L) (n=64) (median (IQR)) | 903 (1410) |
Albumin (g/L) (n=90) (mean ± SD) | 27±5 |
ALAT (μ kat/L) (n=125) (median (IQR)) | 0.52 (0.59) |
- | Survivors, n=85, 57M/28 F | Non-survivors, n=45, 26M/19F | P-value |
Age (median (IQR)) | 66 (19) | 76 (16) | <0.001 |
Hypertension, n(%) | 53(62%) | 36(80%) | 0.047 |
Ischemic heart disease, n(%) | 19(22%) | 20(44%) | 0.015 |
Renal disease, n(%) | 4(5%) | 10(22%) | 0.005 |
Bronchial asthma, n(%) | 12(14%) | 1(2%) | 0.033 |
Haemoglobin (g/L)(median (IQR)) | 139 (25) | 125 (26) | <0.001 |
Troponin (ng/L) (median (IQR)) |
14 (24) | 35 (37.5) | <0.001 |
Creatinine (µmol/L) (median (IQR)) |
88 (40) | 112 (89) | <0.001 |
eGFR (MDRD) (median (IQR)) |
72 (38) | 51 (39) | <0.001 |
Albumin (g/L) (mean ± SD) |
28±5 | 25±5 | 0.034 |
Cough at presentation, n (%) | 62(73%) | 22(48%) | 0.014 |
Gastroenterological symptoms at presentation n (%) | 20(24%) | 2(4%) | 0.005 |
Days from first symptom to admission (median (IQR)) | 7 (5) | 4 (5) | <0.001 |
M=male, F=female |
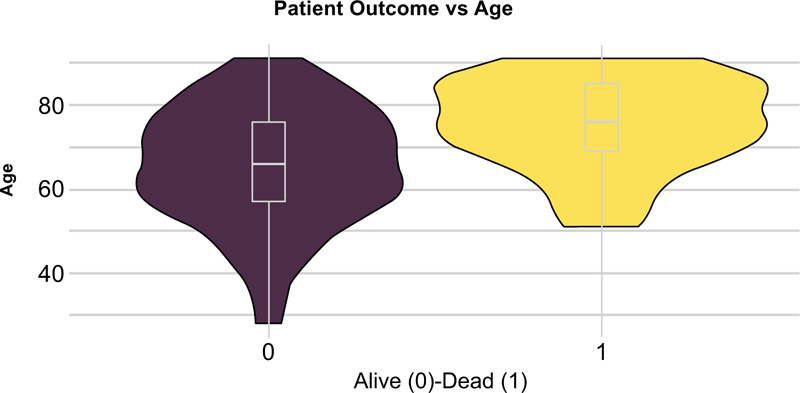
The statistically significant differences between hospital survivors and nonsurvivors are given in Table 2. Compared to the survivors, non-survivors were older (Fig. 2) and had a higher incidence of hypertension, ischemic heart disease, and renal disease (Fig. 3), while diabetes mellitus was not significantly associated with fatal outcomes in our study (p=0.28). Body mass index (BMI) was available in 123 patients, and despite widespread comorbidity with nearly 80% of patients being overweight or obese (Table 1), neither weight status nor BMI was associated with death at a hospital (p=0.38, p=0.45 respectively). Bronchial asthma was more common among hospital survivors, although this correlation was weak related to the small number of patients with asthma diagnosis (Table 2). Cough and gastroenterological symptoms at presentation were more commonly reported among hospital survivors (Table 2). Lower haemoglobin estimated glomerular filtration rate (eGFR), albumin and higher troponin and creatinine levels were the laboratory markers that differed significantly between survivors and nonsurvivors (Table 2) while lymphocytopenia was not found to be significantly associated with in-hospital mortality (p=0.61). Patients who survived had, on average, a longer period from symptom onset to hospital admission (Table 2), (Fig. 4). Patients in group 3 (oxygen treatment) with a lower fraction of inspired oxygen (FiO2) (L/min) need had a higher hospital survival rate (p<0.001).
The 3 groups of patients evaluated one year after COVID-19 infection were examined for differences. No differences in age, BMI and smoking status, lung, or renal disease were found at baseline (data not shown). Hypertension and heart failure was a more common diagnosis in group 3 (Fisher test, p=0.018 and p=0.017, respectively).
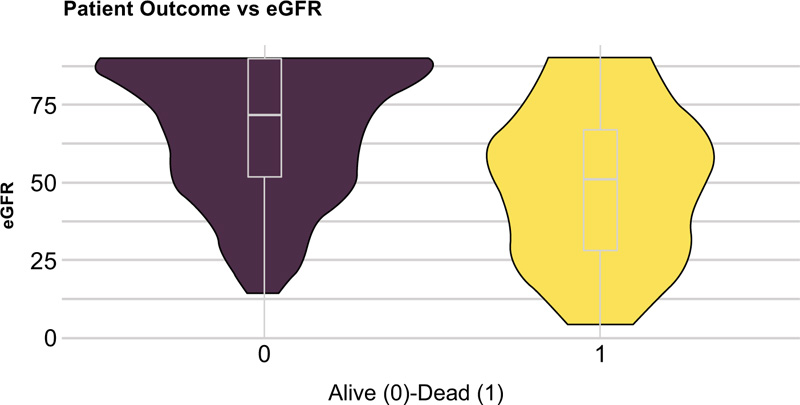
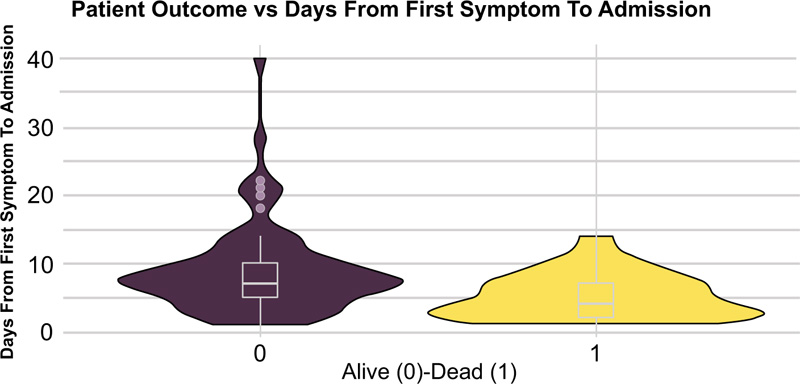
Table 3.
- | ICU/IMV Group 1, n=22 |
HFNC/NIV Group 2, n=17 |
|
---|---|---|---|
FEV1% (mean ± SD) | 86 ± 11 | 85± 16 | p=0.74 |
FVC% (mean ± SD) | 86 ±14 | 84±16 | p=0.70 |
FEV1/FVC (mean ± SD) | 76±9 | 77±8 | P=0.89 |
Scales | ICU/IMV Group 1, n=22 |
HFNC/NIV Group 2, n=18 |
Oxygen treatment Group 3, n=33 |
|
---|---|---|---|---|
HAD-anxiety (mean ± SD) |
6 ±4 | 6 ±5 | 6 ±5 | p=0.84 |
HAD-depression (mean ± SD) | 5±3 | 6±3 | 5±4 | p=0.44 |
PCFS difference (mean ± SD) |
1±0.7 | 1±0.5 | 1±0.6 | p=0.96 |
SF-general health (mean ± SD) |
56±18 | 45±16 | 54±28 | p=0.30 |
mMRC dyspnea scale | ICU/IMV Group 1, n=22 |
HNFC/NIV Group 2, n=18 |
Oxygen treatment Group 3, n=33 |
|
0 | 5 | 5 | 7 | p=0.06 |
1 | 10 | 10 | 8 | |
2 | 7 | 1 | 13 | |
3 | 0 | 2 | 4 | |
4 | 0 | 0 | 1 | |
Pulmonary fibrosis after 1 year |
ICU/IMV Group 1, n=23 |
HNFC/NIV Group 2, n=18 |
Oxygen treatment Group 3, n=33 |
|
0=no | 16 (70%) | 13 (72%) | 19 (58%) | p=0.20 |
1=yes | 6 (26%) | 3 (17%) | 2 (6%) | |
2= has not been examined | 1 (4%) | 2 (11%) | 12 (36%) |
The results of the spirometry in groups 1 (ICU/IMV) and 2 (HFNC/NIV) are shown in Table 3. No statistically significant differences were found between the 2 groups. Mean values of both forced expiratory volume in 1 second (FEV1%) and forced vital capacity (FVC%) indicate that the results from lung function tests performed one year after severe COVID-19 were within the lower normal limits. We did not find any correlation between the maximal oxygen need in FiO2 in group 2 (HFNC/NIV) and spirometry values (p=0.96 for FEV1%, p=0.35 for FVC%).
Table 4 shows the results of the HAD, the PCFS scale, and the SF-36 item. No significant differences were detected in the above scales, including all items of SF-36 among the 3 patient groups.
The distribution of the mMRC dyspnea scale among the 3 groups is also depicted in Table 4. More patients in groups 1 (ICU/IMV) and 2 (HFNC/NIV) suffered from mild dyspnea (mMRC 1), while there was a trend for more significant dyspnea mMRC grade 2 in the third group, although no statistically significant difference was noted between the 3 groups.
Finally, we found that 6 (26%) patients in group 1 (ICU/IMV) had radiological signs of pulmonary fibrosis one year after COVID-19 infection, 3 (17%) patients in group 2 and 2 (6%) patients in group 3 (Table 4). Only one patient was diagnosed with pulmonary fibrosis of idiopathic pulmonary fibrosis/usual interstitial pneumonia (IPF/UIP) before COVID-19 and belonged to group 3. This patient was not included in the analysis (categorised as status 2=fibrosis has not been examined). The number of patients who were not radiologically examined in each group is also shown in (Table 4). We could not identify any differences in the frequency of pulmonary fibrosis between the 3 groups.
4. DISCUSSION
In a rapidly expanding field of knowledge concerning long-term COVID-19 sequelae and heterogeneous patient groups, we contribute demographical data from a population of hospitalised patients. We hypothesized that the initial disease severity could impact the residual function, especially pulmonary. To this end, we divided patients into three groups depending on the reliance of oxygen to keep them saturated as a surrogate marker of pulmonary disease activity. The main result of this study is that the lung function values were at the lower limit of normal when evaluated with dynamic spirometry at one-year follow-up. No significant differences were related to initial disease severity in lung function and long-term health status at 12 months. Increased in-hospital mortality was associated with acute biochemical abnormalities, higher age, heart and renal disease.
Previous studies have shown reduced lung function, radiological abnormalities, and increased rates of depression or anxiety because of COVID-19. Early follow-up at 4-8 weeks showed fatigue (60-72%), dyspnoea (42-65%), and psychological distress (23-46%), more commonly in patients that needed ICU-level care [20]. Similarly, it has been shown that fatigue and dyspnoea were still common at 8-12 weeks, but significant radiological and lung function abnormalities were less frequent, especially in patients without respiratory insufficiency in their acute infection [21]. Huang et al provided large-scale follow-up data from Wuhan, the initial site of the pandemic, showing reduced diffusing capacity for carbon monoxide (DLCO) and radiological abnormalities (primarily ground-glass opacities and irregular lines) at 6 months [10]. There was a correlation between oxygen need and residual pulmonary function and an increased tendency of depression and anxiety [10]. More recent one-year data from the same cohort showed a slight improvement in pulmonary function over time, although diffusion impairment and radiographic abnormalities persisted up to one year, especially in patients who were critically ill during their hospital stay [22]. Another study showed persistent symptoms associated with post-acute COVID-19 syndrome in 67% of patients requiring hospital care compared to 50% of non-admitted patients [23]. One-year radiological follow-up recently showed lingering fibrotic changes with traction bronchiectasis and reticular pattern [24]. One of the most reported abnormalities is a reduced DLCO [8], which by its nature, is difficult to measure in primary care, where most patients with long-lasting symptoms seek medical attention. We focused, therefore, on pulmonary variables that are more easily obtainable, such as dynamic spirometry and health questionnaires.
Collectively, we found a generally reduced, albeit within a reference, pulmonary function with lower FEV1% and FVC%. These observations credit the possibility of long-lasting lung damage even after one year of the infectious event, even though the current evidence is still a bit speculative.
In the studied population, one can note a more common occurrence of comorbidities such as diabetes and obesity compared to the general prevalence [25, 26]. This is an expected finding since these conditions are well-established risk factors for severe COVID-19. We did not see any increased mortality related to diabetes and obesity in this population. Otherwise, we have confirmed previously reported risk factors for mortality during the acute phase, such as hypertension, ischemic heart disease, chronic renal disease, anaemia and the rise of troponin and acute kidney injury [27-29]. Acute kidney injury, in particular, has been identified as highly prognostic of a poor outcome [30]. Evidence suggests a correlation between acute kidney injury during COVID-19 and microthrombotic disease activity [31]. Asthma had a small protective effect, which might seem paradoxical, but has also been reported previously [32]. Perhaps this could be due to inhaled corticosteroids (ICS) treatment, as there has been reported that ICS treatment beneficially alters the prognosis of COVID-19 [33]. Previous reports indicate that prior lung disease, especially advanced COPD, predicts higher mortality [34], which we could not replicate. This could potentially be due to a low prevalence of such patients in our study group, which could imply differences in medical priorities between countries concerning terminal lung disease. The high death toll in the regular oxygen-treated group (group 3) is also heavily skewed due to decisions to withhold advanced life-prolonging treatment in relation to comorbidities.
Contrary to previous data indicating 26% depression and anxiety rates at 1 year in hospitalised patients [22], only 14 patients (11%) reported clinically relevant depression or anxiety based on the HAD SCALE, which is calibrated to rather include than exclude. These results could also be influenced by community restrictions, where Sweden never imposed a formal lock-down. Other studies analyzing non-COVID mental health issues during the pandemic have not found clinically relevant depression and anxiety symptoms or severe psychological distress in general [35], but there are such findings concerning adolescents [36]. And as such, perhaps the higher age in our patient cohort has been a confounding factor. It is also a bit surprising that the prevalence of mental health symptoms is as low as it is compared to reported numbers that reach close to 40% at 1-year follow-up of acute respiratory distress syndrome (ARDS) in the pre-COVID era [37]. Probably, there is a protective element in COVID-19 being recognized as a global pandemic.
Our group's rates of readmissions and subsequent unscheduled medical visits were not correlated to initial disease severity. We noted in addition that even though a large proportion of patients reported dyspnoea as lasting symptoms; many had not done any form of investigation with chest radiology or pulmonary function tests outside of the study; suggesting strain on the health care apparatus to adequately follow up patients and possibly lack of knowledge as well. This also led to relatively few radiological data points, so a robust analysis of prolonged radiological changes was not possible.
The obvious limitation of this study is the relatively small number of participants, but still, almost 64% of all hospitalised patients were ultimately included, providing good regional demographic data. Many patients, specifically those with severe diseases, lived in another geographical location but needed to be transported to our region due to available medical resources. These patients were unfortunately lost to follow-up. Another limitation is the lack of preexisting baseline pulmonary function data. Nonetheless, reduced lung function is not a recognized risk factor for hospital admission [38]. To provide a baseline comparison in the general functional levels, we used the PCFS scale, where patients were asked to grade themselves retrospectively, although the accuracy might have been obscured by the passing of one year.
CONCLUSION
Our results indicate that the long-term prognosis of COVID-19 patients was not influenced greatly by how severe the initial infectious episode was. The lack of difference between the groups with differing acute disease severity suggests that more generous diagnostic manoeuvres, such as spirometry and radiology, could be indicated even in less severely affected individuals with lingering symptoms.
LIST OF ABBREVIATIONS
ALAT | = Alanine Aminotransferase |
ANOVA | = Analysis of Variance |
ARDS | = Acute Respiratory Distress Syndrome |
ATS | = American Thoracic Society |
BMI | = Body Mass Index |
COPD | = Chronic Obstructive Pulmonary Disease |
COVID-19 | = Coronavirus disease 2019 |
CRP | = C-reactive protein |
DLCO | = Diffusing capacity for carbon monoxide |
eGFR | = Estimated glomerular filtration rate |
FEV1 | = Forced expiratory volume in 1 second |
FiO2 | = Fraction of inspired oxygen |
FVC | = Forced vital capacity |
HAD | = Hospital Anxiety and depression scale |
HFNC | = High-flow nasal-cannula |
ICS | = Inhaled corticosteroids |
ICU | = Intensive care unit |
IMV | = Invasive mechanical ventilation |
IQR | = Interquartile range |
MERS | = Middle East Respiratory Syndrome |
mMRC | = Modified Medical Research Council |
NIV | = Non-invasive ventilation |
PCFS | = Post-COVID-19 Functional Status Scale |
PFTs | = Pulmonary function tests |
SARS | = Severe acute respiratory syndrome |
SARS-CoV-2 | = Severe acute respiratory syndrome coronavirus 2 |
SD | = Standard deviation |
SF-36 - | = 36-Item Short-Form Health Survey |
ETHICS APPROVAL AND CONSENT TO PARTICIPATE
The Swedish Ethical Review Authority granted ethical approval for the study (dnr: 2020-03531).
HUMAN AND ANIMAL RIGHTS
No animals were used in this research. All human research procedures followed were per the ethical standards of the committee responsible for human experimentation (institutional and national) and with the Helsinki Declaration of 1975, as revised in 2013.
CONSENT FOR PUBLICATION
Written informed consent was obtained from all living patients.
STANDARDS OF REPORTING
STROBE guideline has been followed.
AVAILABILITY OF DATA AND MATERIALS
The datasets used and/or analysed during the current study are available from the corresponding author on reasonable request.
FUNDING
None.
CONFLICT OF INTEREST
The authors declare that they have no competing interests.
ACKNOWLEDGEMENTS
On behalf of all authors, we would like to thank our colleagues Bertil Ekman, MD, Ph.D. and Magnus Wijkman, MD, Ph.D. for their guidance and constructive comments on the manuscript. We would also like to express our greatest appreciation to the intensive care unit (ICU) staff of Vrinnevi Hospital for their titanic work throughout the pandemic.